Research Article
Volume 3 Issue 2 - 2021
Transfer factors of nickel and Iron levels in the different parts of oil palm (Elaeis guineensis) and their habitat topsoils from Lekir, Peninsular Malaysia
1Department of Biology, Faculty of Science, Universiti Putra Malaysia, 43400 UPM Serdang, Selangor, Malaysia
2Inti International University, Persiaran Perdana BBN, 71800 Nilai, Negeri Sembilan, Malaysia
3All Cosmos Bio-Tech Holding Corporation, PLO650, Jalan Keluli, Pasir Gudang Industrial Estate, 81700 Pasir Gudang, Johor, Malaysia
4MES SOLUTIONS, 22C-1, Jalan BK 5A/2A, Bandar Kinrara, 47100 Puchong, Selangor, Malaysia
5Department of Microbiology, Faculty of Biotechnology and Biomolecular Sciences, Universiti Putra Malaysia, 43400 UPM Serdang, Selangor, Malaysia
6Laboratory of Vaccines and Biomolecules, Institute of Bioscience, Universiti Putra Malaysia, 43400 UPM Serdang, Selangor, Malaysia
7Faculty of Agriculture Universiti Putra Malaysia, 43400 UPM Serdang, Selangor, Malaysia
8Fisheries Research Institute, Batu Maung, 11960 Pulau Pinang, Malaysia
2Inti International University, Persiaran Perdana BBN, 71800 Nilai, Negeri Sembilan, Malaysia
3All Cosmos Bio-Tech Holding Corporation, PLO650, Jalan Keluli, Pasir Gudang Industrial Estate, 81700 Pasir Gudang, Johor, Malaysia
4MES SOLUTIONS, 22C-1, Jalan BK 5A/2A, Bandar Kinrara, 47100 Puchong, Selangor, Malaysia
5Department of Microbiology, Faculty of Biotechnology and Biomolecular Sciences, Universiti Putra Malaysia, 43400 UPM Serdang, Selangor, Malaysia
6Laboratory of Vaccines and Biomolecules, Institute of Bioscience, Universiti Putra Malaysia, 43400 UPM Serdang, Selangor, Malaysia
7Faculty of Agriculture Universiti Putra Malaysia, 43400 UPM Serdang, Selangor, Malaysia
8Fisheries Research Institute, Batu Maung, 11960 Pulau Pinang, Malaysia
*Corresponding Author: Chee Kong Yap, Department of Biology, Faculty of Science, Universiti Putra Malaysia, 43400 UPM Serdang, Selangor, Malaysia
Received: July 28, 2021; Published: August 28, 2021
Abstract
The different parts (fruits, leaves, fronds and barks) of oil palm tree (Elaeis guineensis) and their habitat topsoil collected from four sampling sites in Lekir, Sitiawan, were determined for Ni and Fe. There is no consistent patterns of Ni and Fe distributions between the different parts of the oil palm and their habitat topsoils. The levels of Ni and Fe in the topsoils followed the sampling sites of housing area> factory> road side> forest. However, these metal levels in the different parts of OP do not follow the above order of sites based the topsoil results. For the topsoil sample, the present ranges of Fe (2586-4544 mg/kg) is higher than some reported studies for agriculture soils from Malaysia, while Ni (1.20-4.10 mg/kg) is lower the reported OP soils from Jengka. Based on the Ni transfer factor, two sites exceeded 1.0. However, for Fe transfer factor mean values of all plant parts from all sites are below 1.0. This indicated the capacity of the plant to bioaccumulate Ni, but hardly for Fe, from the soils.
Keywords: Oil Palm; Metal distribution
Introduction
Previously, Yap et al. (2019, 2020) had studied the ability of oil palm (OP), Elaeis guineensis, to accumulate and distribute Cu, Fe, Pb and Zn based on different types of topsoil in their respective habitats. In this paper, the transfer factors of Ni and Fe in the OP based on the same research methodology as reported by Yap et al. (2019, 2020).
The elevated levels of Ni were found to be accumulated in the upper organic horizon of polluted soil (Chang et al. 1984), which might be attributed to the high cation exchange capacity of the surface organic layer (Koen 2013). The living plants are also known for their ability in uptake of heavy metals (Bansode et al. 2003). The uptake of Fe and Ni in plants from soil are mainly influenced by the concentration gradients caused by selective ion uptake of the roots, or by diffusion of elements in the soil. More specific knowledge about the potential of E. guineensis in absorbing and accumulate heavy metals in their different parts are needed in order to understand the accumulation capability of Ni and Fe.
According to Khatun et al. (2017), the plantations and expansion of OP has brought upon detrimental effects on the environment which is disruptive towards the natural flow of the ecosystem as well as increasing the emission of greenhouse gases causing annual fires as well as subsidence and flood risk. The presence of heavy metals in soil environments is known to cause severe damage to living organism. Wuana and Mbasugh (2013) stated that although soils have natural ability to mitigate metals’ toxicity but at elevated levels, this ability is greatly attenuates.
This study aims to determine the transfer factors of Ni and Fe concentrations in the different parts of E. guineensis collected from Lekir, Sitiawan Felcra Berhad Plantation in Perak.
Materials and Methods
The study area, Lekir, Sitiawan Felcra Berhad Plantation (Figure 1) is managed by Felcra Berhad Gugusan Lekir. The Lekir, Sitiawan Felcra Berhad Plantation is located in Manjung, Perak. The samples of E. guineensis were taken from four sampling sites within the plantation namely near a Housing Area (HA), near to a Road Side (RS), near to a small fragmented Forest area (Forest) and an area near to an OP processing Factory (Factory) (Yap et al., 2019).
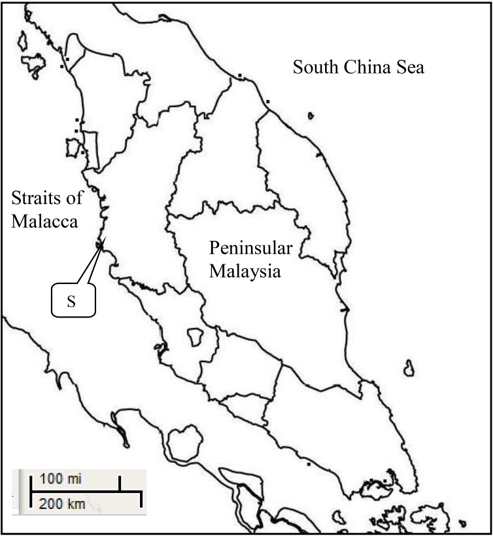
Figure 1: Map showing the sampling sites (S) at Lekir, Sitiawan Felcra Berhad Plantation, Perak, Peninsular Malaysia (Cited from Yap et al., 2019).
Samples of OP parts and their habitat topsoil were collected from the selected sampling sites, in 2014. The separated plant parts included seed, shell, fibre, Leaf Base (LB), Leaf Middle (LM), Leaf Tip (LT), frond, Frond Middle (FM), Frond Right (FR) and bark.
All samples collected were kept and tightly sealed in clean plastic bags to prevent contamination. The samples were brought back to laboratory within one day. All collected OP parts were removed from dust and extraneous matters, such as airborn pollutants, by washing them with distilled water. Samples were then cut down into smaller size and accordingly to their parts. Samples were then dried in oven (60°C) for 3 days before they were grinded and homogenized. The grinded samples were then stored in sealed plastic bags until further digestion.
Soil samples were collected at 6 m width from the OP tree and at 5-10 cm depths. The samples were stored in plastic bags and brought to laboratory. Soil samples were dried in oven at 60°C for 12 hours and were then grinded and homogenized. The soil samples were then sieved with a 75 µm mesh size sieve.
For plant samples, approximately 0.50 g (triplicates) was weighed, placed into digesting tube and later on digested with 10 ml of nitric acid (HNO3). For soil samples, approximately 0.50 g (triplicates) was weighed and placed into digesting tube. However, the acid used for soil digestion were a combination of nitric acid (69%) and perchloric acid (60%) at a ratio of 4:1. Sample digestion were conducted in a heating block digester.
For the first one hour, samples were digested on heating block at 40°C and the last three hours the temperature was raised up to 140°C to ensure all samples were fully digested. The samples were allowed to cool down and were later diluted by adding 40 ml of distilled water. Then all samples were filtered through Whatman No.1 filter paper into pillboxes. Procedural blank is prepared in order to check for sample accuracy.
Working standards of different concentrations of Ni and Fe were prepared from standard solutions. The concentrations of Ni and Fe were determined by using an air-acetylene flame atomic absorption spectrometer (AAS), Perkin Elmer model Analyst 800. Analysis of certified reference materials (CRMs) namely,NCS DC73319-Soil and MESS-3-Soil were used to verify the analytical procedures. Their recoveries were obtained and were found to be in an acceptable range between 90.4 and 96.7% (Table 1).
Table 1: Comparisons of Ni and Fe concentrations (µg/g dry weight) between certified reference materials (CRM) values and the measured values, and their recoveries.
Metals | Samples | CRM values | Measured value | Recovery Percentage (%) |
Ni | NCS DC73319-Soil | 20.40 | 19.5 ± 1.74 | 102 ± 0.82 |
MESS-3-Soil | 46.9 ± 2.2 | 40.93 ± 6.23 | 94.64 ± 1.10 | |
Fe | NCS DC73319-Soil | NA | NA | NA |
MESS-3-Soil | 4.34 ± 0.11 | 5.06 ± 3.45 | 88.12 ± 1.23 |
Note: NA= not available.
It should be noted that different parts of the same plant species displayed different heavy metals’ enrichment abilities. In order to understand the exchange of heavy metals between the soil and OP, the transfer factor (TF) was calculated based on the formula suggested by Li et al. (2012):
TF= Cplant/Csoil
Where Cplant = metal concentration in the plant (mg/kg); Csoil = metal concentration in soil.
Where Cplant = metal concentration in the plant (mg/kg); Csoil = metal concentration in soil.
Results and Discussion
The distributions of Ni in the different parts of E. guineensis for four sampling sites at are presented in Table 2. The levels of Ni in the topsoils are HA> Fact> RS> Forest. However, for the OP parts, all OP parts collected from RS site have the highest concentrations of Ni (P< 0.05). The Ni levels in the ten different parts from RS site range from 14.5 to 24.6 mg/kg dry weight which are significantly higher when compared to the other three sites that range from 0.70 to 4.30 mg/kg dry weight (Table 2). The disagreement of Ni levels between the OP habitat topsoils and different OP parts could be due to the bioavailable Ni in the topsoils to the OP uptake is dependent on the pH of the soils. However, this has become an interesting topic for further studies.
Table 2: Distributions of concentrations (mg/kg dry weight) of Ni and Fe in the different parts of Elaeis guineensis collected from four sampling sites in Perak, Peninsular Malaysia.
Ni | Seed | Shell | Fibre | Leaf (Base) | Leaf (Middle) | Leaf (Tip) | Frond (Left) | Frond (Middle) | Frond (Right) | Bark | Soil |
HA | 2.80 | 1.90 | 0.30 | 2.10 | 2.60 | 2.30 | 1.80 | 1.20 | 3.20 | 1.50 | 4.10 |
RS | 14.5 | 18.5 | 20.1 | 20.7 | 23.4 | 24.6 | 23.6 | 23.5 | 23.8 | 22.5 | 2.60 |
Forest | 2.50 | 4.30 | 1.70 | 2.20 | 2.20 | 1.20 | 1.80 | 3.00 | 1.80 | 1.90 | 1.20 |
Fact | 1.50 | 0.70 | 1.10 | 2.30 | 1.00 | 1.80 | 2.20 | 2.90 | 0.90 | 0.90 | 3.60 |
Fe | Seed | Shell | Fibre | Leaf (Base) | Leaf (Middle) | Leaf (Tip) | Frond (Left) | Frond (Middle) | Frond (Right) | Bark | Soil |
HA | 11.8 | 8.30 | 19.2 | 78.5 | 74.5 | 77.6 | 4.70 | 26.3 | 67.6 | 134 | 4544 |
RS | 59.2 | 74.3 | 80.1 | 184 | 179 | 183 | 84.9 | 76.1 | 81.8 | 120 | 3098 |
Forest | 29.8 | 30.5 | 36.1 | 119 | 103 | 102 | 20.1 | 26.1 | 26.2 | 71.9 | 2586 |
Fact | 31.4 | 36.5 | 29.0 | 220 | 263 | 306 | 38.0 | 17.8 | 34.9 | 66.6 | 3528 |
Note: HA= near housing area; RS= near roadside; Fact= near a palm oil processing factory. Values in bold are the highest among the four sites.
The distributions of Fe in the different parts of E. guineensis for four sampling sites at are also presented in Table 2. Similarly, the levels of Fe in the topsoil are HA> Fact> RS> Forest. Only OP bark was found to record the highest Fe levels in HA, when compared to the other three sites. RS site recorded the highest Fe levels in seeds, shell, fibre, leaf frond, middle frond and right frond. The highest levels of Fe for factory were recorded in LB, LM and LT. These results are difficult to interpret since many factors could play the roles in the uptake of Fe to the different parts of the OP.
Yap et al. (2019) reported the levels of Cu in the topsoils are Factory> HA> RS> Forest. For the plant parts, the highest levels of Cu are also significantly (P< 0.05) found in all the plant parts collected from Factory site. The levels of Pb in the topsoil are RS> Forest> Factory> HA. For the plant parts, the highest levels of Pb are significantly (P< 0.05) found in the bark, frond, FM, FL and shell collected from RS site. Whereas, the highest levels of Pb are consistently found the LT, LM, LB, fibre, and seeds collected from HA.
The present Fe range (2586-4544 mg/kg) is higher than the Fe contents (2302 mg/kg) in agricultural soils from Besut (Malaysia) (Khairiah et al. 2012) and OP soils from Jengka (136 mg/kg) (Sulaiman et al. 2016). Also, the present Ni OP soils (1.20-4.10 mg/kg) are lower the OP soils from Jengka (14.0 mg/kg) (Sulaiman et al. 2016). This may be due to the different s agronomic practices and environmental factors. The levels also depend on the natural concentration of Ni and Fe in the habitat topsoils.
Being the key component of Earth’s curst, Fe mainly formed the sedimentary rock in soil in the form of oxide or hydroxide (Mico et al. 2006). The increased of Fe levels exceeding 100 mg/kg compared to other metals could be attributed to the use of fertilizers in the agriculture sector. On the other hand, the sources of Ni could be inputs from natural sources and anthropogenic activities. The main sources of anthropogenic Ni were from sewage sludge (Cempel and Nikel 2006) and fertilizer (Tang et al. 2014). The common usage of organic fertilizers to increase the growth of crops could be the reason of high Ni levels in agricultural soils.
The metal ranges for Ni and Fe in the habitat topsoils of all four sampling sites are 1.20 to 4.10 mg/kg dry weight, and ; 2586 to 4544 mg/kg dry weight, respectively. The metal ranges are lower than the Ni (40.0 mg/kg) maximum allowable concentrations in the China Environmental Quality Standards for Soil (GB 15618-1995, grade II for the agriculture land) (Liu et al., 2011), and Fe background value (30900 mg/kg) (Wedepohl 1995).
Transfer Factor
The ratios of OP (different parts) to soils for Ni and Fe in the four sampling sites are given in Table 3. Based on the TF mean Ni values of all OP parts, the sites from RS and Forest exceeded 1.00, but not for HA and Factory sites (Table 3). Based on the TF mean Fe values of all OP parts, all the four sites are below 1.00. Therefore, the use of different parts of OP to remove Fe from the soils is not promising.
The ratios of OP (different parts) to soils for Ni and Fe in the four sampling sites are given in Table 3. Based on the TF mean Ni values of all OP parts, the sites from RS and Forest exceeded 1.00, but not for HA and Factory sites (Table 3). Based on the TF mean Fe values of all OP parts, all the four sites are below 1.00. Therefore, the use of different parts of OP to remove Fe from the soils is not promising.
Table 3: Ratios of Elaeis guineensis (different parts) to soils for Ni and Fe in the four sampling sites at Lekir, Sitiawan Felcra Berhad Plantation area, Perak, Malaysia.
Ni | Seed | Shell | Fibre | Leaf (Base) | Leaf (Middle) | Leaf (Tip) | Frond (Left) | Frond (Middle) | Frond (Right) | Bark |
HA | 6.83E-01 | 4.63E-01 | 7.32E-02 | 5.12E-01 | 6.34E-01 | 5.61E-01 | 4.39E-01 | 2.93E-01 | 7.80E-01 | 3.66E-01 |
RS | 5.58E+00 | 7.12E+00 | 7.73E+00 | 7.96E+00 | 9.00E+00 | 9.46E+00 | 9.08E+00 | 9.04E+00 | 9.15E+00 | 8.65E+00 |
Forest | 2.08E+00 | 3.58E+00 | 1.42E+00 | 1.83E+00 | 1.83E+00 | 1.00E+00 | 1.50E+00 | 2.50E+00 | 1.50E+00 | 1.58E+00 |
Fact | 4.17E-01 | 1.94E-01 | 3.06E-01 | 6.39E-01 | 2.78E-01 | 5.00E-01 | 6.11E-01 | 8.06E-01 | 2.50E-01 | 2.50E-01 |
Fe | Seed | Shell | Fibre | Leaf (Base) | Leaf (Middle) | Leaf (Tip) | Frond (Left) | Frond (Middle) | Frond (Right) | Bark |
HA | 2.60E-03 | 1.83E-03 | 4.22E-03 | 1.73E-02 | 1.64E-02 | 1.71E-02 | 1.03E-03 | 5.79E-03 | 1.49E-02 | 2.95E-02 |
RS | 1.91E-02 | 2.40E-02 | 2.59E-02 | 5.95E-02 | 5.77E-02 | 5.90E-02 | 2.74E-02 | 2.46E-02 | 2.64E-02 | 3.88E-02 |
Forest | 1.15E-02 | 1.18E-02 | 1.40E-02 | 4.58E-02 | 3.99E-02 | 3.94E-02 | 7.77E-03 | 1.01E-02 | 1.01E-02 | 2.78E-02 |
Fact | 8.90E-03 | 1.03E-02 | 8.22E-03 | 6.24E-02 | 7.44E-02 | 8.67E-02 | 1.08E-02 | 5.05E-03 | 9.89E-03 | 1.89E-02 |
Yap et al. (2019) reported the TF mean Cu values of all plant parts, the sites from RS, Forest and Factory exceeded 1.0, but not for HA. In fact, all the plant parts from Factory site exceeded 1.0. This indicated the capacity of the plant to bioaccumulate Ni and Cu (Yap et al., 2019) from the soils. Yap et al (2019) reported that the TF mean Pb values of all plant parts, only all the parts from HA exceeded 1.00 while RS, Forest and Factory are below 1.00.
Therefore, the use of different parts of OP in the removal of Ni, Cu and Pb from the habitat topsoils are potential under the phytoremediation technique in order to reduce the ecological risk and/or removal of heavy metals from contaminated soils (Khare et al. 2016).
The distribution of metal concentrations found in the different OP parts is related to the different functions of each part in the OP (Yap et al., 2019). Since the age of E. guineensisin this study is approximately 20 years, this indicated that the trees are in a stable condition for nutrients uptake as well as the heavy metals uptake from the soil. Wang et al. (2005) has reported that the soil pH may change due to long term application of chemical fertilizers.
The highest levels of Fe were found in topsoils and tree bark (but not for Fe). According to Emilsson (2008), bark protects the interior tissues of a tree from external physical threats. Bark also serves as a dumping ground in which the tree rids itself of waste products from its metabolism.
Ni is commonly found in organically bound form and their bioavailability and mobility are increased acidic and neutral conditions. Albeit, there were no evidence that Ni is an essential element for optimal growth of plants, it is easily available, especially at pH < 6 (Kabata-Pendias and Pendias, 1999).
Conclusion
The levels of Ni and Fe in the topsoils followed the sampling sites of HA> Fact> RS> Forest. However, these metal levels in the different parts of OP do not follow the above order of sites. Based on the Ni TF mean values of all plant parts, the sites from RS and Forest exceeded 1.0 (1.53-7.53). This indicated the capacity of the OP parts to bioaccumulate Ni from the soils. However, the Fe TF mean values of all OP parts are all below 1.0. The present finding indicated that different parts of E. guineensis is a potential bioaccumulator in the removal of Ni from their surrounding soil due to high TF values of Ni in the different parts of OP collected from the two sites.
Acknowledgement
The authors wish to acknowledge the partial financial support provided through the Fundamental Research Grant Scheme (FRGS), [Vote no.: 5524953], by Ministry of Higher Education, Malaysia.
The authors wish to acknowledge the partial financial support provided through the Fundamental Research Grant Scheme (FRGS), [Vote no.: 5524953], by Ministry of Higher Education, Malaysia.
Conflict of Interest
The authors declare that there are no conflicts of interest.
The authors declare that there are no conflicts of interest.
References
- Bansode, R.R., Losso, J.N., Marshall, W.E., Rao, R.M. and Portier, R.J. (2003). Adsorption of metal ions by pecan shell-based granular activated carbons. J. Biores. Technol., 89: 115–119.
- Cempel, M. and Nikel, G. (2006). Nickel: a review of its sources and environmental toxicology. Pol. J. Environ. Stud., 3: 373–382.
- Chang, A.C., Warnecke, J.E., Lund, L.J., and Page, A.L. (1984). Accumulation of heavy metals in sewage sludge-related soils. J. Environ. Qual., 13(1): 87-91.
- Emilsson, T. (2008). Vegetation development on extensive vegetated green roofs: in?uence of substrate composition, establishment method and species mix. Ecol. Eng., 33: 265–277.
- Kabata-Pendias, A. and Pendias, H. (1999). Biogeochemistry of trace elements. 2nd ed. Warsaw: PWN.
- Khairiah, J., Tharmendren, M.S.M., Habibah, J., Zulkefly, H., Wan Kamal, W.I. and Ismail, B.S. (2012). Heavy metal content in paddy soils of Ketara, Besut, Terengganu, Malaysia. World Appl Sci J., 19(2): 183–191
- Khare, S., Khare, R. and Jaya. (2016). Removal of toxic heavy metals from biosphere by plants. Int. J. Chem. Sci. 14(3): 1541–1548.
- Khatun, R., Reza, M.I.H., Moniruzzaman, M., and Yaakob, Z. (2017). Sustainable oil palm industry: The possibilities. Renew. Sustain. Energy Rev. 76:608–619.
- Koen, O. (2013). Heavy metals in soils: Trace metals and metalloids in soils and their bioavailability. Environ. Pollut. 13:367–368.
- Li, Q., Chen, Y., Fu, H., Cui, Z., Shi, L., Wang, L., and Liu, X. (2012). Health risk of heavy metals in food crops grown on reclaimed tidal flat soil in the Pearl River Estuary, China. J. Hazard. Mater. 227–228: 148–154.
- Liu, J., Zhang, X-H., Tran, H., Wang, D-Q. and Zhu, Y-N. (2011). Heavy metal contamination and risk assessment in water, paddy soil, and rice around an electroplating plant. Environ. Sci. Pollut. Res., 18: 1623–1632.
- Loska, K., Wiechul, D. and Korus, I. (2004). Metal contamination of farming soils affected by industry. Environ. Int., 30: 159– 165
- Mico, C., Retacala, L., Peris, M. and Sanchez, J. (2006). Assessing heavy metal sources in agriculture soil of a European Mediterranean are by multivariate analysis. Chemosphere 65: 863–887.
- Sulaiman, F.R., Mustaffa, N.F.S. and Mohd Khazaai, S.N. (2016). Preliminary assessment of selected metals in agricultural soils in Jengka, Pahang, Malaysia. Environ. Earth Sci., 75: 223.
- Tang, W., Ao, L., Zhang, H. and Shan, B. (2014). Accumulation and risk of heavy metals in relation to agricultural intensification in the river sediments of agricultural regions. Environ. Earth Sci., 71: 3945–3951.
- Wang, S.Q., Zhou, D.M., Wang, Y.J. and Chen, H.M. (2005). Nutrient variation in plastic greenhouse soils with the years of cultivation. Soil (in Chinese), 37: 460–462.
- Wedepohl, K.H. (1995). The composition of the continential crust. Geochim. et Cosmochim. Acta, 59: 1217–1232.
- Wuana, R.A. and Mbasugh, P.A. (2013). Responses of roselle (Hibiscus sabdariffa) to heavy metals contamination in soils with different organic fertilizations. Chem. Ecol., 29(5): 437–447.
- Yap, C.K., Nur Aishah, H., Cheng, W.H., Zakaria, M. P. and Al-Shami, S.A. (2019). Bioaccumulation of Cu and Pb in the different parts of oil palm (Elaeis guineensis) in comparison to their habitat topsoils. In: Soil Pollution: Sources, Management Strategies and Health Effects, Editor: Chee Kong Yap, Nova Science Publishers, New York, USA.
- Yap, C.K., Cheng, W.h., Nur Aishah, H., Tony Peng, S.H., Ibrahim, M.H., Nulit, N., Yap, C.W., Sharifinia, M., Riyahi Bakhtiari, A., Al-Shami, S.A., Leow, C.S., and Saupi Ismail, M. (2020). A preliminary study on the status of essential Cu, Fe and Zn in the leaflets of oil palm (Elaeis guineensis) collected from Lekir, Peninsular Malaysia. ES Journal of Nutritional Health 1(1): 110.
Citation: Chee Kong Yap., et al. (2021). Transfer factors of nickel and Iron levels in the different parts of oil palm (Elaeis guineensis) and their habitat topsoils from Lekir, Peninsular Malaysia. Journal of Agriculture and Aquaculture 3(2).
Copyright: © 2021 Chee Kong Yap. This is an open-access article distributed under the terms of the Creative Commons Attribution License, which permits unrestricted use, distribution, and reproduction in any medium, provided the original author and source are credited.