Review Article
Volume 1 Issue 1 - 2019
Vaccine Production in Transgenic Plants for Animal and Human Diseases
1College of Veterinary Medicine and Agriculture, Addis Ababa University
2National animal health diagnostic and investigation Center (NAHDIC)
2National animal health diagnostic and investigation Center (NAHDIC)
*Corresponding Author: Fanos Tadesse woldemariyam, College of Veterinary Medicine and Agriculture, Addis Ababa University.
Received: September 04, 2019; Published: September 13, 2019
Abstract
Vaccines are a biological preparation that provides active acquired immunity to a particular disease. They can be produced by using different methods of which the egg-based vaccines, cell-based vaccines, and vaccines produced using investigational-manufacturing (plant, bacterial culture and insect cell) systems are some to mention. The technology of the use of plants as a bioreactor to produce human or animal therapeutic vaccines receives increasing attention. Many of these subunit vaccines have been purified and also administered orally in a non-purified form as a food or feed product and this review mainly focuses on edible vaccines. Antigens from several human and veterinary pathogens have been expressed in transgenic plants, including Norwalk virus, rabies, measles, hepatitis B, anthrax, infectious bursal disease virus, and avian influenza virus. Production of edible subunit-based recombinant vaccine proteins in the form of leaves, seeds or fruit is expected to be cost effective, and products will be easily stored and transported under limited refrigeration without degradation. Administration of commercial edible vaccines will require significantly less labor and technical training of medical and veterinary personnel. Despite these promising attributes, there still remain concerns and challenges with edible vaccine development, such as achieving maximum expression levels, possible immune tolerance and allergy, as well as cross contamination concerns. Edible plant-derived vaccines may lead to a future of safer and more effective immunization practice.
Keywords: Bioreactor; Immunization; Non-purified; Purified; Transgenic Plants; Vaccine
Introduction
Vaccines are a biological preparation that provides active acquired immunity to a particular disease by stimulating the antibodies production in animal and humans against several diseases. Egg-based vaccines, cell-based vaccines, and vaccines produced using investigational-manufacturing systems are some methods to mention (Doshi et al., 2013). The egg-based vaccine produced in 9-to-12 day-old embryonated eggs have some drawbacks such as lengthy process, inability to replicate in embryonated eggs and large number of eggs are required (Greer, 2015). Whereas cell based vaccine uses high priced fermentation technology. So, vaccination represents one of the most cost-efficient tools to combat and eradicate diverse pathogenic and/or infectious agents spread around the world (Gomez et al., 2010).
Owing to these limitations other alternative investigational-manufacturing system, which utilizes plant, bacterial culture, or insect cells, have recently gained the attention of researchers (Penney et al., 2011). Of which plant-based vaccine production has received particular attention due to the numerous advantages it may offer. Plant can be used as bioreactor (transgenic plants which can be used to produce the proteins or peptides encoded by the introduced foreign genes) in vaccine production against animal and human diseases. Recently advances in plant genetic engineering technology have changed the concept of growing crops from food supply to a bioreactor for the production of therapeutic recombinant proteins and plant-based vaccines Here plants are used as bioreactors to express antigen proteins triggered by plant transgenic vectors to produce vaccines. As eukaryotic organisms, plants can synthesize, express, and process complex heterologous proteins similarly to conventional, fermentation-based expression systems (Rybicki, 2010).
The first demonstration of expression of a vaccine antigen within plants occurred in 1990 when Curtiss and Cardineau expressed the Streptococcus mutans surface protein antigen A (SpaA) in tobacco (Curtiss andCardineau,1990) followed by plant expression of the hepatitis B surface antigen and the E. coli heat-labile enterotoxin responsible for diarrhea (Walmsley and Arntzen,2000). Livestock have been fed transgenic plants like alfalfa, expressing antigens to protect them from various pathogens, including foot-and-mouth disease virus (FMDV), bovine rotavirus (BRV) and bovine viral diarrhea virus (BVDV) (Wu et al., 2004). To the best of our knowledge there are limited written materials regarding plant based vaccine development and application Therefore the objective of this review paper is to review on plant based vaccine, method of development and its application in both human and veterinary medicine.
Developing Process Of Plant Based Vaccine
Careful selection of one or more immune protective antigens and designing of genes and promoters that would express the antigen(s) at a high level in target plant tissue followed by genetic transformation and foreign antigen extraction and Immunogenicity assay are the key steps in the development process(Floss et al., 2007) (Figure 1).
Careful selection of one or more immune protective antigens and designing of genes and promoters that would express the antigen(s) at a high level in target plant tissue followed by genetic transformation and foreign antigen extraction and Immunogenicity assay are the key steps in the development process(Floss et al., 2007) (Figure 1).
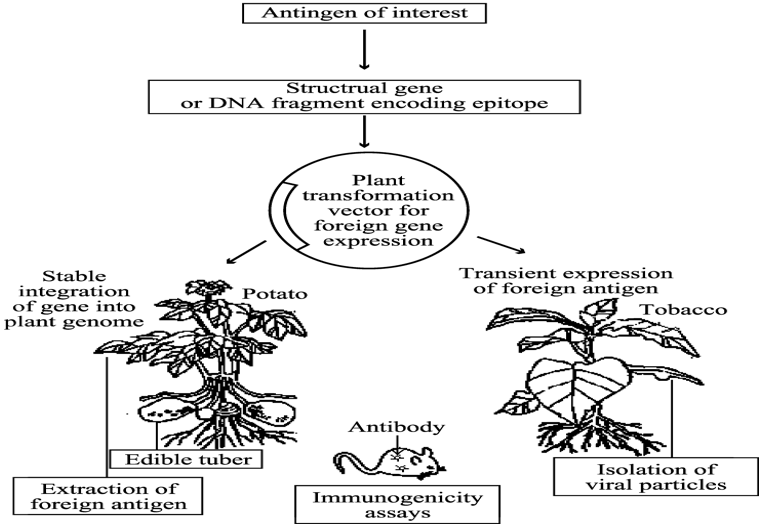
Source: Adapted from Mason and Arntzen, 1995
Figure 1: Process of producing transgenic plant vaccines.
Figure 1: Process of producing transgenic plant vaccines.
Selection of the Desired Gene
The underlying reason that transgenic plants can be constructed is the universal presence of DNA (deoxyribonucleic acid) in the cells of all living organisms. Genetic information is specified by the sequence of four chemical bases (adenine, cytosine, guanine, and thymine) along the length of the DNA molecule. Genes are discrete segments of DNA that encode the information necessary for assembly of a specific protein. The proteins then function as enzymes to catalyze biochemical reactions, as structural or storage units of a cell for contributed expression of a plant trait (Yoshi Matsu, 2012).
The underlying reason that transgenic plants can be constructed is the universal presence of DNA (deoxyribonucleic acid) in the cells of all living organisms. Genetic information is specified by the sequence of four chemical bases (adenine, cytosine, guanine, and thymine) along the length of the DNA molecule. Genes are discrete segments of DNA that encode the information necessary for assembly of a specific protein. The proteins then function as enzymes to catalyze biochemical reactions, as structural or storage units of a cell for contributed expression of a plant trait (Yoshi Matsu, 2012).
Plant Selection
The level of expression, processing, storage, type, delivery methods and the major diet of the target individual are the basis to select a particular plant (Daniell et al., 2001). Different plants have been demonstrated for production of recombinant proteins in plants, including leafy crops/plants, dry seed crops, fruits and vegetables (Fischer et al., 2004). The best plant used for vaccine should have expression in edible tissue that can be consumed uncooked, easy for transformation amenability, target tissue to be rich in protein, target tissue should not produce toxic molecules, correct folding of the antigen protein and desired post-translational modifications should be allowed (Floss et al., 2007).
The level of expression, processing, storage, type, delivery methods and the major diet of the target individual are the basis to select a particular plant (Daniell et al., 2001). Different plants have been demonstrated for production of recombinant proteins in plants, including leafy crops/plants, dry seed crops, fruits and vegetables (Fischer et al., 2004). The best plant used for vaccine should have expression in edible tissue that can be consumed uncooked, easy for transformation amenability, target tissue to be rich in protein, target tissue should not produce toxic molecules, correct folding of the antigen protein and desired post-translational modifications should be allowed (Floss et al., 2007).
Vegetables
Some vegetables are used as the receptor of plant-derived vaccines because many of them are appetizing, free of toxicant, full of nutrient and freshly edible. Potato (Solanumtuberosum), tomato (Lycopersiconesculentum) and carrot (DaucuscarotasubspeciesSativus) have already been reported to successfully express vaccine candidates (Walmsley and Arntzen, 2000). The main problem here is low expression level (Koprowski, 2005).
Some vegetables are used as the receptor of plant-derived vaccines because many of them are appetizing, free of toxicant, full of nutrient and freshly edible. Potato (Solanumtuberosum), tomato (Lycopersiconesculentum) and carrot (DaucuscarotasubspeciesSativus) have already been reported to successfully express vaccine candidates (Walmsley and Arntzen, 2000). The main problem here is low expression level (Koprowski, 2005).
Leafy crops/plants
Tobacco (Nicotianatabacum) and Alfalfa (Medic ago sativa) are the two major leafy crops used for the production of recombinant proteins. Both have high leaf biomass yields in part because they can be cropped several times every year. The main limitation is limited shelf life for the harvested plant. This can decrease the product yield because these recombinant proteins exist in an aqueous environment and are relatively unstable (Conrad and Fiedler, 1998).
Tobacco (Nicotianatabacum) and Alfalfa (Medic ago sativa) are the two major leafy crops used for the production of recombinant proteins. Both have high leaf biomass yields in part because they can be cropped several times every year. The main limitation is limited shelf life for the harvested plant. This can decrease the product yield because these recombinant proteins exist in an aqueous environment and are relatively unstable (Conrad and Fiedler, 1998).
Dry seed crops
Dry seed crops that have been used as host plants for molecular farming include the cereals maize, rice, wheat and barley and the grain legume soybean, pea, pigeon pea and peanut. Maize, rice, wheat, barley, soybean and pea have been investigated as general production platforms, while pigeon pea and peanut have been used only for the expression of animal vaccine candidates. The major advantage of all seed crops are that the accumulation of recombinant proteins specifically in the dry seeds. Therefore, although seed biomass yields are smaller than the leaf biomass yields of tobacco and alfalfa; this is balanced by the increased stability of the proteins (Sack et al., 2000). Seeds are natural storage organs, with the optimal biochemical environment for the accumulation of large amounts of protein. In the best cases, recombinant proteins expressed in seeds have been shown to remain stable and active after storage at room temperature for over three years. The accumulation of proteins in the seed rather than vegetative organs also prevents any toxic effects on the host plant. Finally, the extraction of proteins from seeds is easy because the target protein is concentrated in a small volume part of the plant. Additionally most cereal seeds lack the phenolic compounds which interfere with processing. The seed proteome is also simple product which will co-purify with the recombinant protein during downstream processing (Vaquero et al., 2000).
Dry seed crops that have been used as host plants for molecular farming include the cereals maize, rice, wheat and barley and the grain legume soybean, pea, pigeon pea and peanut. Maize, rice, wheat, barley, soybean and pea have been investigated as general production platforms, while pigeon pea and peanut have been used only for the expression of animal vaccine candidates. The major advantage of all seed crops are that the accumulation of recombinant proteins specifically in the dry seeds. Therefore, although seed biomass yields are smaller than the leaf biomass yields of tobacco and alfalfa; this is balanced by the increased stability of the proteins (Sack et al., 2000). Seeds are natural storage organs, with the optimal biochemical environment for the accumulation of large amounts of protein. In the best cases, recombinant proteins expressed in seeds have been shown to remain stable and active after storage at room temperature for over three years. The accumulation of proteins in the seed rather than vegetative organs also prevents any toxic effects on the host plant. Finally, the extraction of proteins from seeds is easy because the target protein is concentrated in a small volume part of the plant. Additionally most cereal seeds lack the phenolic compounds which interfere with processing. The seed proteome is also simple product which will co-purify with the recombinant protein during downstream processing (Vaquero et al., 2000).
Fruits
Banana (Musa acuminate) is one of the earliest fruits used for plant transgenic programs. Promoter MaExp1 is considered as an important tool for expressing foreign proteins (vaccine) in banana fruit at the time of ripening (Mason et al., 2002). Papaya (Carica papaya) is also a widespread tropical and semi-tropical fresh edible fruit which is important to produce vaccine (Sciutto et al., 2002). In these cases no need of cooking which is an important advantage of using them to protect heat sensitive vaccines (Lal et al., 2007).
Banana (Musa acuminate) is one of the earliest fruits used for plant transgenic programs. Promoter MaExp1 is considered as an important tool for expressing foreign proteins (vaccine) in banana fruit at the time of ripening (Mason et al., 2002). Papaya (Carica papaya) is also a widespread tropical and semi-tropical fresh edible fruit which is important to produce vaccine (Sciutto et al., 2002). In these cases no need of cooking which is an important advantage of using them to protect heat sensitive vaccines (Lal et al., 2007).
Selection of Promoter
This is the most important part for successful genetic transformation and the expression of gene. Coding region of gene with sustainable promoter can increase and adjust the expression level and patterns of foreign gene. Promoters act as a gene switches which turn on the genes so that to determine when, where, how much and how for these genes were remained turned on. A complex intercommunication network was formed from these gene conversations that facilitate the tens of thousands of genes to work in a consistent expression mode in an organism (Shinozaki et al., 2003). Based on their activities promoters can be of constitutive, tissue specific and inducible promoters. Constitutive promoters are used when the expression of gene is required all the time and anywhere in the plant. The cauliflower mosaic (CaMV) 35S and maize ubiquitin-1 promoter (ubi-1), for dicots and monocots, respectively are common plant promoter that is used for constitutive over expression of specific genes(Fischer et al., 2004).Tissue specific promoters are used to control gene expression in particular plant tissue at particular developmental stage. Promoters from E8 gene and French bean b-phase Olin gene one are most commonly used fruit and seed specific promoter respectively (Nicholas et al., 1995; Vander et al., 1997).
This is the most important part for successful genetic transformation and the expression of gene. Coding region of gene with sustainable promoter can increase and adjust the expression level and patterns of foreign gene. Promoters act as a gene switches which turn on the genes so that to determine when, where, how much and how for these genes were remained turned on. A complex intercommunication network was formed from these gene conversations that facilitate the tens of thousands of genes to work in a consistent expression mode in an organism (Shinozaki et al., 2003). Based on their activities promoters can be of constitutive, tissue specific and inducible promoters. Constitutive promoters are used when the expression of gene is required all the time and anywhere in the plant. The cauliflower mosaic (CaMV) 35S and maize ubiquitin-1 promoter (ubi-1), for dicots and monocots, respectively are common plant promoter that is used for constitutive over expression of specific genes(Fischer et al., 2004).Tissue specific promoters are used to control gene expression in particular plant tissue at particular developmental stage. Promoters from E8 gene and French bean b-phase Olin gene one are most commonly used fruit and seed specific promoter respectively (Nicholas et al., 1995; Vander et al., 1997).
Whereas inducible promoters are used for expression of gene at certain condition activated by various stimuli like plant hormones, chemicals and various abiotic and biotic stresses. The maize inducible 2-2 and peroxidase gene promoters can be mentioned as an example here (Veylder et al., 1997; Ma et al., 2003).
Gene insertion and Expression System
Four methods of gene expression have been used so far for the purpose of recombinant protein production in plants. These are stable nuclear transformation of a crop species (field or a greenhouse grown) stable plastid transformation of a crop species, transient transformation of a crop species and stable transformation of a plant species (Nikolov and Hammes, 2002).
Four methods of gene expression have been used so far for the purpose of recombinant protein production in plants. These are stable nuclear transformation of a crop species (field or a greenhouse grown) stable plastid transformation of a crop species, transient transformation of a crop species and stable transformation of a plant species (Nikolov and Hammes, 2002).
The Stable nuclear transformationmethod involves the incorporation of a foreign gene of interest into the nuclear genome of the plant. This alters the genetic makeup and leads to the expression of the transgene after integration with the host genome. This system requires a method for transferring the foreign genes into the plant cells, usually using Agro-bacterium tumefaciens or particle bombardment, in which the genes are taken up and incorporated into the host nuclear genome in a stable manner(Horn et al., 2004) (Figure 2).
The Stable plastid transformation is another method of introducing DNA into the chloroplast genome, usually by particle bombardment (Figure 3) and Agro-bacterium tumefaciens (Figure 4). This system provides a valuable alternative to nuclear transformation because of no cross pollination problem as plastids are inherited through maternal tissues in most species, hence the transgene may not be transferable, thereby alleviating public concerns. The limitation of this method is fresh tissue molecular farming system protein stability over time will change even with refrigeration. Therefore extraction and purification must be performed at very specific times following harvest (Meyers et al., 2010).
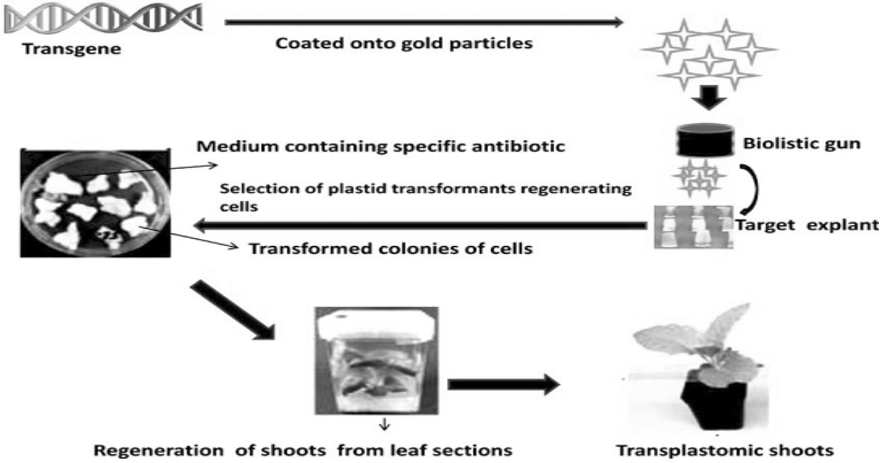
Source: https://www.ncbi.nlm.nih.gov/pmc/articles/PMC3048312
Figure 3: Stable plastid transformation by using particle bombardment.
Figure 3: Stable plastid transformation by using particle bombardment.
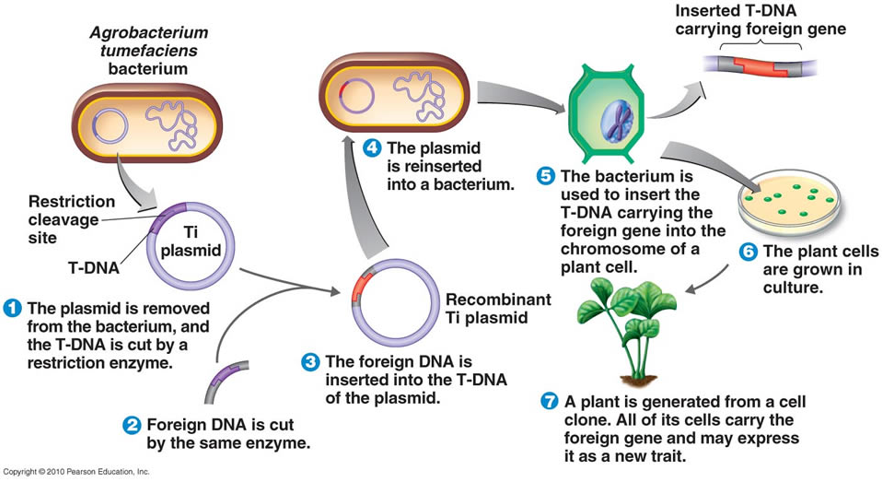
Source: https://futurism.com/genetic-engineering-a-primer
Figure 4: Stable plastid transformation using Agro-bacterium.
Figure 4: Stable plastid transformation using Agro-bacterium.
The Transient expression system is perhaps the fastest and the most convenient production platform for plant molecular farming (Rybicki, 2010).The most commonly used expression methods are Agro infiltration, Virus infection and the magnification technology (Vezina et al., 2009).
The agro-infiltration method involves infiltration of a suspension of recombinant Agro-bacterium tumefaciens into tobacco leaf tissue, which facilitates the transfer of T-DNA to a very high percentage of the cells, where it expresses the transgene at very high levels. This method has now been developed into a very rapid, high-yielding transient expression strategy for producing clinical grade bio-pharmaceuticals (Vezina et al., 2009; Pogue et al., 2010). The virus infection method is dependent on the ability of plant viruses such as tobacco mosaic virus (TMV) and potato virus X (PVX) to be used as vectors to deliver foreign genes into plants, without integration (Porta and Lomonossoff, 2002).
Production and Delivery
Currently there are two investigations directed to transgenic plant vaccines production. The first is the use of plants to produce plentiful foreign antigen protein, and then manufacturing into vaccines after separation and purification. The second one is direct use of fresh edible plants or plant tissues to congregate the interest protein as oral/edible vaccines without isolation and purification (Lamp hear et al., 2004).
Currently there are two investigations directed to transgenic plant vaccines production. The first is the use of plants to produce plentiful foreign antigen protein, and then manufacturing into vaccines after separation and purification. The second one is direct use of fresh edible plants or plant tissues to congregate the interest protein as oral/edible vaccines without isolation and purification (Lamp hear et al., 2004).
Purification of Plant-Based Vaccines
The high cost of pharmaceutical protein production is because of the difficulty of purification of the recombinant proteins. There are many problems for purification of recombinant proteins in plants, such as influence of polyphenols, alkaloids, quinonoid compounds and some other secondary metabolites. Therefore novel recombinant protein purification strategies, which do not require the application of expensive column chromatography, may be used. For example: The use of a protein-based polymer with inverse temperature transition properties to purify pro-insulin expressed by chloroplast vectors via simple centrifugation is a major accomplishment (Daniell et al., 2005). Foreign proteins expressed in seeds assure excellent storage properties and thus added flexibility in processing management and batch production (Baez et al., 2000). Alternative methods including the use of oleo sin or polymer fusions to facilitate purification of recombinant proteins may also be applicable to antibody molecules (Schillberg et al., 2005) and finally the expensive step of purifying recombinant proteins could be eliminated if the protein assembled exclusively at edible tissues (tomato, potato, banana, and papaya etc). Oral delivery of the recombinant proteins could cut down 90% of the production costs (Daniell et al., 2001).
The high cost of pharmaceutical protein production is because of the difficulty of purification of the recombinant proteins. There are many problems for purification of recombinant proteins in plants, such as influence of polyphenols, alkaloids, quinonoid compounds and some other secondary metabolites. Therefore novel recombinant protein purification strategies, which do not require the application of expensive column chromatography, may be used. For example: The use of a protein-based polymer with inverse temperature transition properties to purify pro-insulin expressed by chloroplast vectors via simple centrifugation is a major accomplishment (Daniell et al., 2005). Foreign proteins expressed in seeds assure excellent storage properties and thus added flexibility in processing management and batch production (Baez et al., 2000). Alternative methods including the use of oleo sin or polymer fusions to facilitate purification of recombinant proteins may also be applicable to antibody molecules (Schillberg et al., 2005) and finally the expensive step of purifying recombinant proteins could be eliminated if the protein assembled exclusively at edible tissues (tomato, potato, banana, and papaya etc). Oral delivery of the recombinant proteins could cut down 90% of the production costs (Daniell et al., 2001).
Oral Delivery, Mucosal and Systemic Antibody Responses
Delivery by needles is a particular problem in developing countries, where safe injection practices cannot be guaranteed, especially when needles are reused. Plant based vaccines can be- delivered orally or via an intranasal spray without needles, thus making vaccines cheaper, safer, and more efficacious. As most infectious agents enter the body through mucosal membranes induction of mucosal immunity is best achieved by direct oral vaccine delivery to mucosal surfaces because edible vaccines come in contact with the lining of the digestive tract. This stimulates production of IgA, the predominant antibody isotope in mucosal secretion (Walmsley et al., 2000).
Delivery by needles is a particular problem in developing countries, where safe injection practices cannot be guaranteed, especially when needles are reused. Plant based vaccines can be- delivered orally or via an intranasal spray without needles, thus making vaccines cheaper, safer, and more efficacious. As most infectious agents enter the body through mucosal membranes induction of mucosal immunity is best achieved by direct oral vaccine delivery to mucosal surfaces because edible vaccines come in contact with the lining of the digestive tract. This stimulates production of IgA, the predominant antibody isotope in mucosal secretion (Walmsley et al., 2000).
The induction of immunity is notoriously difficult to achieve through injections, which by pass mucosal barriers. In part, this accounts for the reason as to why the efficacy of injectable vaccines against intestinal pathogens has generally been quite poor. Edible vaccines also have positive implications for livestock welfare, as they could be administered directly in the diet, eliminating the need to confine the animal, or the need to breach the skin through injection, a practice that can promote secondary infections. Oral administration of vaccines eliminates the risks that injection-based methods pose on meat quality as a result of intramuscular administration. The major obstacle to oral vaccination is the digestion of the antigenic protein in the stomach. Vaccines derived and delivered by plant cells have been shown to overcome this problem through the protective effect of the plant cell wall. Like liposomes and microcapsules, the plant cell wall allows gradual release of the antigen onto the vast surface area of the lower digestive tract (Sala et al., 2003).
Application
Plant based Oral vaccines are potentially applicable to any vaccine formulation based on or including a subunit component. Plants allow for the rapid bulk up of large supplies of subunit vaccines, for example a 40,000-fold increase per year is possible using corn. Therefore, oral vaccines are particularly applicable to combating diseases that affect very large populations. Furthermore, oral plant-based vaccines are stable during storage at ambient temperatures and do not require syringes, needles and trained personnel for administration. These features also favour the use of oral vaccines for large-scale immunization programmes, particularly in developing countries with limited resources to provide a cold chain and the equipment and personnel needed for injections and to protect against sudden outbreaks of disease in developed countries as a result of, terrorist actions. This can have both in animals and human application which are discussed below (Lamphear et al., 2002).
Plant based Oral vaccines are potentially applicable to any vaccine formulation based on or including a subunit component. Plants allow for the rapid bulk up of large supplies of subunit vaccines, for example a 40,000-fold increase per year is possible using corn. Therefore, oral vaccines are particularly applicable to combating diseases that affect very large populations. Furthermore, oral plant-based vaccines are stable during storage at ambient temperatures and do not require syringes, needles and trained personnel for administration. These features also favour the use of oral vaccines for large-scale immunization programmes, particularly in developing countries with limited resources to provide a cold chain and the equipment and personnel needed for injections and to protect against sudden outbreaks of disease in developed countries as a result of, terrorist actions. This can have both in animals and human application which are discussed below (Lamphear et al., 2002).
Application in Veterinary Medicine
The concept of using plants as expression hosts for veterinary subunit vaccines has been studied extensively within the past two decades. Below are some vaccines used in veterinary medicine:-
The concept of using plants as expression hosts for veterinary subunit vaccines has been studied extensively within the past two decades. Below are some vaccines used in veterinary medicine:-
Plant based vaccine against poultry diseases
An edible potato-based vaccine against chicken infectious bronchitis virus was developed by sliced tubers expressing viral S1 glycoprotein (IBV). Orally immunized chickens developed a virus-specific antibody response and were protected against IBV (Zhou et al., 2004). Another vaccine was prepared against infectious bursal disease virus (IBDV) with plant made VP2 protein. The chickens were orally immunized with Arabidopsis crude leaf extracts or transgenic rice seeds and protected to a similar level achieved with a commercial injectable vaccine (Gomez et al., 2013). For Newcastle disease plant cell culture based vaccine have been tried and approved by FDA in 2006. This vaccine was composed of recombinant hem-agglutinin-neuraminidase protein expressed in transgenic tobacco suspension cells and formulated as an injectable vaccine. Other studies on Newcastle disease virus include expression of full-size glycoproteins in transgenic potato and tobacco leaves, maize and rice seeds. The antigens were shown to be immunogenic and protective in chicken safer oral delivery (Joensuu et al., 2008).
An edible potato-based vaccine against chicken infectious bronchitis virus was developed by sliced tubers expressing viral S1 glycoprotein (IBV). Orally immunized chickens developed a virus-specific antibody response and were protected against IBV (Zhou et al., 2004). Another vaccine was prepared against infectious bursal disease virus (IBDV) with plant made VP2 protein. The chickens were orally immunized with Arabidopsis crude leaf extracts or transgenic rice seeds and protected to a similar level achieved with a commercial injectable vaccine (Gomez et al., 2013). For Newcastle disease plant cell culture based vaccine have been tried and approved by FDA in 2006. This vaccine was composed of recombinant hem-agglutinin-neuraminidase protein expressed in transgenic tobacco suspension cells and formulated as an injectable vaccine. Other studies on Newcastle disease virus include expression of full-size glycoproteins in transgenic potato and tobacco leaves, maize and rice seeds. The antigens were shown to be immunogenic and protective in chicken safer oral delivery (Joensuu et al., 2008).
Plant based vaccine against swine diseases
Plant based vaccine have been used for various swine diseases. A maize seed as an edible delivery vehicle against porcine Transmissible gastroenteritis corona virus (TGEV) has been used. In this case envelope spike protein was used as an antigen to raise neutralizing antibodies of piglets (Lamp hear et al., 2004). Post-weaning diarrhea in piglets caused by Enterotoxigenic E. coli (ETEC) has been also a target for a plant-made vaccine by expressing subunit protein of ETEC F4 fimbriae in the leaves of tobacco alfalfa and in seeds of barley and showed immunogenicity and partially protective after oral delivery to weaned piglets (Kolotilin et al., 2012). Another plant based vaccine was also prepared by using capsid protein VP1 antigenic epitope of the of Foot and- mouth disease virus (FMDV) which is propagated in plants by using bamboo mosaic virus coat protein. It was demonstrated that immunization with the extracted chimeric virions by intramuscular injection could fully protect pigs against FMDV challenge by effectively inducing hum oral and cell-mediated immune responses (Yang et al., 2007).
Plant based vaccine have been used for various swine diseases. A maize seed as an edible delivery vehicle against porcine Transmissible gastroenteritis corona virus (TGEV) has been used. In this case envelope spike protein was used as an antigen to raise neutralizing antibodies of piglets (Lamp hear et al., 2004). Post-weaning diarrhea in piglets caused by Enterotoxigenic E. coli (ETEC) has been also a target for a plant-made vaccine by expressing subunit protein of ETEC F4 fimbriae in the leaves of tobacco alfalfa and in seeds of barley and showed immunogenicity and partially protective after oral delivery to weaned piglets (Kolotilin et al., 2012). Another plant based vaccine was also prepared by using capsid protein VP1 antigenic epitope of the of Foot and- mouth disease virus (FMDV) which is propagated in plants by using bamboo mosaic virus coat protein. It was demonstrated that immunization with the extracted chimeric virions by intramuscular injection could fully protect pigs against FMDV challenge by effectively inducing hum oral and cell-mediated immune responses (Yang et al., 2007).
Plant based vaccine against cattle diseases
Immunogenicity of a Tobacco mosaic virus (TMV)-based vaccine against bovine herpes virus (BHV) (Perez et al., 2003), the structural protein E2 of BVDV in transgenic alfalfa (Aguirreburualde et al., 2013), and that of B. anthracisin transgenic tobacco, potato and tomato plant shave been developed by different researchers in different corners of the world. These can have the ability to act at both humoral and cell mediated immune system depending on the type of vaccines (Azizet al., 2005).
Immunogenicity of a Tobacco mosaic virus (TMV)-based vaccine against bovine herpes virus (BHV) (Perez et al., 2003), the structural protein E2 of BVDV in transgenic alfalfa (Aguirreburualde et al., 2013), and that of B. anthracisin transgenic tobacco, potato and tomato plant shave been developed by different researchers in different corners of the world. These can have the ability to act at both humoral and cell mediated immune system depending on the type of vaccines (Azizet al., 2005).
Application in Human Medicine
There are many plant based vaccine developed toward human pathogen of which some of them are described below: Cholera toxin B subunit (CTB) of V. cholera is a plant based vaccine which is responsible for inducing both mucosal and serum immunity. Since the cholera toxin is internalized by the receptors present on mucosal lining, the CTB was one of the early toxins selected for testing the concept of edible vaccines. Here nuclear transformation of tobacco plant was reported using Agrobacterium tumefaciens carrying a gene encoding the CTB engineered with a sequence specific for an endoplasmic reticulum localization signal (SEKDEL) under the control of CaMV35S promoter (Jani et al., 2004).
There are many plant based vaccine developed toward human pathogen of which some of them are described below: Cholera toxin B subunit (CTB) of V. cholera is a plant based vaccine which is responsible for inducing both mucosal and serum immunity. Since the cholera toxin is internalized by the receptors present on mucosal lining, the CTB was one of the early toxins selected for testing the concept of edible vaccines. Here nuclear transformation of tobacco plant was reported using Agrobacterium tumefaciens carrying a gene encoding the CTB engineered with a sequence specific for an endoplasmic reticulum localization signal (SEKDEL) under the control of CaMV35S promoter (Jani et al., 2004).
Recently an Ebola virus subunit vaccine has been generated using a plant Gemini virus-based expression vector. The construct was attained by fusing the glycoprotein (GP1) of Ebola virus to the C terminus of the heavy chain of an IgmAb that is specific for GP1 (Phoolcharoen et al., 2011). Tobacco leaves that expressed the vector containing this construct produced a fully assembled IgG molecule that could be harvested and purified. The purified immunoglobulin was injected into mice and generated an antibody response comparable to that produced with a VLP. This vaccine has also been generated in lettuce (Lai et al., 2012).
The Human papilloma-virus HPV type 11 (HPV11) L1 major capsid protein coding sequence inserted into potato plants was used to develop transgenic potato plant and fed to mice which resulted in an activation of anti-VLP immune response that was qualitatively similar to that induced by VLP parental administration. This immune response in mice was enhanced significantly by subsequent oral booster with purified insect cell-derived VLPs expressed HPV11 L1major capsid protein in transgenic A. thaliana and N. tabacum (Kohl et al., 2007).
Advantages and Limitations of plant Based Vaccine
Plant based edible vaccines have many advantages over the injected vaccines like those edible vaccines are cost and time effective, in many cases; it costs significantly less to grow plants with the ability to produce pharmaceutical proteins in large quantities because plant-based techniques requires soil rich land instead of machineries and does not need cold chain storages. Plant-made pharmaceutical production can be more easily expanded to provide larger quantities if demand for the vaccine increase. Additionally low cost for medical equipment, Medical professionals are not a must, low contamination risks (No chi et al., 2007), multi-component ability (allow for several antigens to approach M cells (micro fold cells) simultaneously and good efficiency to provoke a mucosal immune are among the advantages of edible vaccines (Lal et al., 2007).
Plant based edible vaccines have many advantages over the injected vaccines like those edible vaccines are cost and time effective, in many cases; it costs significantly less to grow plants with the ability to produce pharmaceutical proteins in large quantities because plant-based techniques requires soil rich land instead of machineries and does not need cold chain storages. Plant-made pharmaceutical production can be more easily expanded to provide larger quantities if demand for the vaccine increase. Additionally low cost for medical equipment, Medical professionals are not a must, low contamination risks (No chi et al., 2007), multi-component ability (allow for several antigens to approach M cells (micro fold cells) simultaneously and good efficiency to provoke a mucosal immune are among the advantages of edible vaccines (Lal et al., 2007).
The limitations are low level of expression of heterologous proteins , proper dosage problem, it may trigger immune tolerance, Cross pollination of transgenic plant to other food crops, Gene silencing issue and effects on insects and soil microbes are the major once that hinder the progress (Tiwari and Verma, 2009; Desai,2010).
Conclusion and Recommendations
Plants have the potential to rapidly produce recombinant proteins on a large scale at a relatively low cost compared to other production systems. The basic advantage of using plants as vaccine production system is that plants being higher eukaryotes provide opportunities for unlimited production, the range and diversity of recombinant molecules namely peptides, polypeptides and complex multimeric proteins that cannot be made in microbial systems. On all accounts, the application of plant expression systems to produce human or animal vaccines have a lot of advantages, such as low cost of production, well-established cultivation, rapid scale-up, simple distribution by seeds, ease of genetic manipulation, oral delivery and low-health risks from human pathogen and toxin contamination, etc. so using plant-derived vaccines may lead to a future of safer and more effective immunization practice.
Based on the above concluding remarks the following recommendations are suggested.
- Research should be done in developing Plant based vaccine in countries like Ethiopia.
- Research centers and vaccine production centers should focus on this area as it is very use full as compared to the other methods.
- High expression level should be gained by using novel promoters and increasing protein stability.
- Higher institutions teaching veterinary medicine and human medicine should incorporate related courses in the curriculum.
- Issues, such as the biosafety, uniformity of dosage, the regulatory guidelines, the potential presence of herbicide, and pesticide residues should be seen carefully.
References
- Aguirreburualde, M., Gomez, M., Ostachuk, A., Wolman, F., Albanesi, G., Pecora, A., Odeon, A., Ardila, F., Escribano, J., Dus-Santos, M. and Wigdorovitz, A. (2013): Efficacy of a BVDV subunit vaccine produced in alfalfa transgenic plants. Veterinary Immunolgy and Immuno-pathology 151: 315–324.
- Aziz, M., Sikriwal, D., Singh, S., Jarugula, S., Kumar, P. and Bhatnagar, R. (2005): Transformation of an edible crop with the pagA gene of Bacillus anthracis. Journal of Federation of American Societies for Experimental Biology 19: 1501–1530.
- Baez, J., Russell, D. and Craig, J. (2000): Corn seed production of therapeutic proteins moves forward, one company’s experience. Biopharmaceuticals 13: 50-54
- Conrad, U. and Fiedler, U. (1998): compartment-specific accumulation of recombinant immunoglobulins in plant cells: an essential tool for antibody production and immunomodulation of physiological functions and pathogen activity, Plant Molecular Biology 38: 101–109
- Curtiss, R. and Cardineau, C. (1990): Oral immunisation by transgenic plants, World Patent Application WO 90/02484. Proceeding of National Academy of Science USA 95: 2481-2485.
- Daniell, H., Chebolu, S., Kumar, S., Singleton, M. and Falconer, R. (2005): Chloroplast-derived vaccine antigens and other therapeutic proteins. Vaccine 23: 1779-1783.
- Daniell, H., Streatfield, S. and Wycoff, K. (2001): Medical molecular farming: production of antibodies, biopharmaceuticals and edible vaccines in plants. Trends in Plant Science 6: 219-226.
- Desai, P., Shrivastava, N. and Padh, H. (2010): Production of heterologous proteins in plants: strategies for optimal expression. Biotechnology Advance 28: 427-435.
- Doshi, V., Rawal, H. and Mukherjee, S. (2013): Edible vaccines from GM crops: current status and future scope, Journal of Pharmaceutical and Scientific Innovation 2:1–6.
- Fischer, R., Stoger, E., Schillberg, S., Christou, P. and Twyman, R. (2004): Plant-based production of biopharmaceuticals. Current Opinion in Plant Biology, 7: 2152-158.
- Floss, D., Falkenburg, D. and Conrad, U. (2007): Production of vaccines and therapeutic antibodies for veterinary applications in transgenic plants: an overview. Transgenic Research 16: 315–332.
- Gomez, E., Lucero, M., ChimenoZoth, S., Carballeda, J., Gravisaco, M. and Berinstein, A. (2013): Transient expression of VP2 in Nicotiana benth amiana and its use as a plant-based vaccine against infectious bursal disease virus. Vaccine 31: 2623–2627.
- Gomez, E., Zoth, S., Carrillo, E. and Berinstein, A. (2010): Developments in plant-based vaccines against diseases of concern in developing countries. Journal of the Open Infectious Disease 4: 55-62.
- Greer, A. (2015): Early vaccine availability represents an important public health advance for the control of pandemic influenza, Medical Center Research Notes 8:191
- Horn, M., Woodard, S. and Haward, J. (2004): Plant molecular farming; systems and products. Plant cell reports 22: 711-720.
- Jani, D., Singh, N., Bhattacharya, S., Meena, L., Singh Y. and Upadhyay, S. (2004): Studies on the immunogenic potential of plant-expressed cholera toxin B subunit. Plant Cell Reports 22:471–477.
- Joensuu, J., Niklander-Teeri, V. and Brandle, J. (2008): Transgenic plants for animal health: Plant-made vaccine antigens for animal infectious disease control. Phytochemical Review, 7: 553–577.
- Kohl, T. O., Hitzeroth, I.I., Christensen, N. D. and Rybicki, E.P. (2007): Expression of HPV-11 L1 protein in transgenic Arabidopsis thaliana and Nicotianatabacum. Biomedical Center Biotechnology 7: 56 -69.
- Kolotilin, I., Kaldis, A., Devriendt, B., Joensuu, J., Cox, E. and Menassa, R. (2012): Production of a subunit vaccine candidate against porcine post-weaning diarrhea in high-biomass trans plastomic tobacco. Public Library of Science 7: 42-45.
- Koprowski, H. (2005): Vaccines and sera through plant biotechnology. Vaccine 23: 1757 -1763.
- Lai, H., He, J., Engle, M., Diamond, M. and Chen, Q. (2012): Robust production of virus-like particles and monoclonal antibodies with geminiviral replicon vectors in lettuce. Journal of Plant Biotechnology 10: 95–104.
- Lal, P., Ramachandran, V., Goyal, R. and Sharma, R. (2007): Edible vaccines: Current status and future. Indian Journal of Medical Microbiology 25: 93-102.
- Lamphear, B., Jilka, J., Kesl, L., Welter, M., Howard, J. and Streatfield, S. (2004) Acorn-based delivery system for animal vaccines: an oral transmissible gastroenteritis virus vaccine boosts lactogenic immunity in swine. Vaccine 22: 2420–2424.
- Lamphear, B., Streatfield, S., Jilka, J., Brooks, C., Barker, D., Turner, D., Delaney, D., Garcia, M., Wiggins, W., Woodard, S., Hood, E., Tizard, I., Lawhorn, B. and Howard, J. (2002): Delivery of subunit vaccines in maize seed. Journal of Controlled Release 85: 169–180.
- Ma, J., Drake, P. and Christou, P. (2003): The production of recombinant pharmaceutical in plants. Nature Reviews Genetics 4: 794-805.
- Mason, H.and Arntzen, C. (1995): Transgenic plants as vaccine production systems. Trends in biotechnology 13: 388-392.
- Mason, H., Warzecha, H., Mor, T. and Arntzen, C. (2002): Edible plant vaccines: applications for prophylactic and therapeutic molecular medicine. Trends in Molecular Medicine 8: 324-329.
- Meyers, B., Zaltsman, A., Lacroix, B., Kozlovsky, S. and Krichevsky, A. (2010): Nuclear and plastid genetic engineering of plants: comparison of opportunities and challenges. Biotechnology Advance 28: 747-756.
- Nicholas, F., Smith, C., Schuch, W., Bird, C. and Grierson, D. (1995): High levels of ripening. Specific reporter gene expression directed by tomato fruit polygalacturonase gene-flanking regions. Molecular Biology 28: 423-435.
- Nikolov, Z. and Hammes, D. (2002): Production of recombinant proteins from transgenic crops.In: Hood EE, Howard JA (eds) Plants as factories for protein production, Kluwer academic publisher, Dordrecht, Netherlands, Pp 159–174.
- Nochi, T., Takagi, H., Yuki, Y., Yang, L., Masumura, T., Mejima, M., Nakanishi, U., Matsumura, A., Uozumi, A. and Hiroi, T. (2007): Rice-based mucosal vaccine as a global strategy for cold-chain and needle-free vaccination. Proceeding of National Academy Science, 104: 10986-10991.
- Penney, C., Thomas D., Deen, S. and Walmsley A. (2011): Plant-made vaccines in support of the Millennium Development Goals, Plant Cell Reports 30: 789–798.
- Perez Filgueira, D., Zamorano, P., Dominguez, M., Taboga, O., Del Medico Zajac, M., Puntel, M., Romera, S., Morris, T., Borca, M. and Sadir, A. (2003): Bovine herpes virus gD protein produced in plants using a recombinant tobacco mosaic virus (TMV) vector possesses authentic antigenicity. Vaccine 21: 4201–4209.
- Phoolcharoen, W., Bhoo, S., Lai, H. and Ma, J. (2011): Expression of an immunogenic Ebola immune complex in Nicotianabenthamiana. Journal of Plant Biotechnology 9: 807–816.
- Pogue, G., Vojdani, F., Palmer, K., HiattHume, S. and Phelps, J. (2010): Production of pharmaceutical-grade recombinant aprotinin and a monoclonal antibody product using plant-based transient expression systems. Journal of Plant Biotechnology 8: 638–654.
- Porta,C. and Lomonossoff, G. (2002): Viruses as vectors for the expression of foreign sequences in plants. Biotechnology Genetic Engineering Review 19: 245–91.
- Rybicki, E. (2010): Plant-made vaccines for humans and animals. Journal of Plant Biotechnology 8: 620–637.
- Sack, M., Stoger, E. and Perrin, Y. (2000): Molecular Farming: plant made pharmaceutical and technical protein In Rainer F. and Stefan S.John Wiley and Sons.inc publisher, Germany 9:149–158.
- Sala, F., Rigano, M., Barbante, A., Basso, B., Walmsley, A. and Castiglione, S. (2003): Vaccine antigen production in transgenic plants: strategies, gene constructs and perspectives. Vaccine 21: 803-808.
- Schillberg, S., Twyman, R. and Fischer, R. (2005): Opportunities for recombinant antigen and antibody expression in transgenic plants technology assessment. Vaccine 23: 1764-1769.
- Sciutto, E., Fragoso, G., Manoutcharian, K., Gevorkian, G., Rosas-Salgad, G.,Hernandez-Gonzalez, M., Herrera-Estrella, L., Cabrera-Ponce, J., Lápez-Casillas, F., González-Bonilla, C., Santigago-Machuca, A., Sánchez, R., Goldbaum, F., Alujac, A. andLarraldea, C. (2002): New approaches to improve peptide vaccine against porcine Taeniasolium Cysticercosis. Archives of Medical Research 33: 371-378.
- Shinozaki, K., Yamaguchi-Shinozaki, K. and Seki, M. (2003): Regulatory network of gene expression in the drought and cold stress responses. Current Opinion in Plant Biology 6: 410-417.
- Tiwari, S., Verma, P., Singh, P. and Tuli, R. (2009): Plants as bioreactors for the production of vaccine antigens. Biotechnology Advance 27: 449-467.
- Vander, A., Geest, M. and Hall, T. (1997): The -phaseolin5 matrix attachment region acts as an enhancer facilitator. Plant Molecular Biology, 33: 553-557.
- Vaquero, C., Stoger, E. and Torres, E. (2000): Molecular Farming: plant made pharmaceutical and technical protein. In: Rainer F. and Steafan S,John Wiley and Sons.inc publisher, Germany 42: 583–590.
- Veylder, L., Van, M. and Inze, D. (1997): Herbicide safener inducible gene expression in Arabidopsis thaliana. Plant Cell Physiology 38: 568-577.
- Vézina, L., Faye, L., Lerouge, P., D'Aoust, M., Marquet-Blouin, E. and Burel, C. (2009): Transient coexpression for fast and high-yield production of antibodies with human-likeN-glycans in plants. Journal of Plant Biotechnology 7: 442–55.
- Walmsley, A. and Arntzen, C. (2000): Plants for delivery of edible vaccines. Current Opinion in Biotechnology 11: 126-129.
- Wu, H., Singh, N., Locy, R., Scissum-Gunn, K. and Giambrone, J. (2004): Immunization of chickens with VP2 protein of infectious bursal disease virus expressed in Arabidopsisthaliana. Avian Diseases 48:663-668.
- Yang, C., Liao, J., Lai, C., Jong, M., Liang, C., Lin, Y., Lin, N., Hsu, Y. and Liang, S. (2007): Induction of protective immunity in swine by recombinant bamboo mosaic virus expressing foot-and-mouth disease virus epitopes. Biomedical Center Biotechnol 7: 62.
- Yoshimatsu, K., Kawano, N., Kawahara, N., Akiyama, H., Teshima. R. and Nishijima, M. (2012): Current status of application and commercialization of genetically modified plants for human and livestock health and phytoremediation. Yakugaku Zasshi 132: 629-674.
- Zhou, J., Cheng, L., Zheng, X., Wu, J., Shang, S., Wang, J. and Chen, J. (2004): Generation of the transgenic potato expressing full-length spike protein of infectious bronchitis virus. Journal of Biotechnology 111: 121–130.
Citation: Birtukan Girma, Dereje Shegu, Ayelech Muluneh and Fanos Tadesse woldemariyam. (2019). Vaccine Production in Transgenic Plants for Animal and Human Diseases. Archives of Veterinary and Animal Sciences 1(1).
Copyright: © 2019 Fanos Tadesse woldemariyam. This is an open-access article distributed under the terms of the Creative Commons Attribution License, which permits unrestricted use, distribution, and reproduction in any medium, provided the original author and source are credited.