Research Article
Volume 2 Issue 2 - 2020
The Neuropathic Pain in Animals
University Centre of Veterinary Medicine JU-AU, Hugon Kollataj University of Agriculture in Krakow, A. Mickiewicza Avenue 24/28, 30-059 Krakow, Poland
*Corresponding Author: Bogdan Feliks Kania, University Centre of Veterinary Medicine JU-AU, Hugon Kollataj University of Agriculture in Krakow, A. Mickiewicza Avenue 24/28, 30-059 Krakow, Poland.
Received: June 14, 2020; Published: June 24, 2020
Abstract
Neuropathic pain is serious therapeutic problem. Current therapy is often ineffective and the available drugs have serious side effects. For these reasons, the search for alternative therapeutic solutions is underway. Recent research on metabotropic receptors for glutamic acid (mGluR) gives great hope for the development of a new type of drug in the treatment of neuropathic pain. Particularly promising are antagonists of mGluR group I receptors. There are numerous studies demonstrating the efficacy of non-competitive mGlu1 and mGlu5 receptor antagonists in animal models of neuropathic pain. The purpose of this review article is to gather information obtained from research on the role of mGluR antagonists in neuropathic pain.
Keywords: Neuropathic pain; Glutamate; mGluR antagonists
Introduction
Pain – a phenomenon encompassing all living beings (including plants – 38) – possesses numerous definitions. One of them defines pain as a comprehensive, multi-dimensional experience engaging sensory and emotional factors. Thus, pain is not only a physical, but also a psychical phenomenon – an unpleasant sensation causing pain-related suffering in a living being. According to the International Association for the Study of Pain (IASP), official definition of pain is „an unpleasant sensory and emotional experience associated with actual or potential tissue damage, or described in terms of such damage” (26). Pain is a subjective experience in humans, animals and plants, which make it hard to assess individually. In speech-deprived beings, including animal and plants, behavioral symptoms and knowledge about potential underlying causes are used for pain assessment. In plants, despite their lack of the nervous system, pain (as a stress factor) elicits release of glutamate (Glu) at the site of action. Glutamate, by stimulating structures similar to glutaminergic receptors of the vertebrates, causes activation of ionotropic Glu receptor (iGluR). This leads to the release of calcium ions cascade, which spreads like waves to cells located far from the site of action of the noxious factor (noxa). Target cells release tissue hormones that activate protective systems (alarming systems), essential for defense against pain/stress (26).
Among various methods of pain classification, the following types are distinguished: acute pain (mild, moderate, intense, very intense), chronic, receptor pain (nociceptive – somatic, visceral – diffuse, projected) and extrareceptor pain termed non-receptor or neuropathic, which is also a chronic pain. The last one doesn't have many experimental references facilitating aid for animals suffering from it. Therefore, the aim of this review article is to convince its readers to modern management in the prevention and treatment of neuropathic pain in animals, especially since treatment of this pain results in obtaining 30-50% effective pain reduction in 50% of patients after using different medications (33, 34).
The definition of neuropathic pain
Neuropathic pain is a specific form of pain caused by primary lesion (injury) or dysfunction in somatosensory part of the central nervous system (CNS) or peripheral nervous system (PNS) and is associated with nerve root injuries or post-traumatic plexus injuries and pathology within the nervous system (1, 25). Every type of chronic pain can evolve into pain with nervous background, due to constant excitation of nociceptive receptors and consecutive changes in the functionality of the nervous system (21).
Neuropathic pain is a specific form of pain caused by primary lesion (injury) or dysfunction in somatosensory part of the central nervous system (CNS) or peripheral nervous system (PNS) and is associated with nerve root injuries or post-traumatic plexus injuries and pathology within the nervous system (1, 25). Every type of chronic pain can evolve into pain with nervous background, due to constant excitation of nociceptive receptors and consecutive changes in the functionality of the nervous system (21).
Pain associated with osteoarthritis is of both inflammatory and neuropathic nature. It may occur due to nerve damage after surgery, injuries, tumors located in the peripheral nervous system, some spinal diseases and many more. It is often not a consequence of the cause that primarily triggered it, but an alteration occurring in pain conduction pathways (42).
Injury | complex regional pain syndrome, persistent post-operative pain |
Infection | postherpetic neuralgia, HIV-induced neuropathy |
Ischemia | central poststroke pain |
Cancer | compression or infiltration of nervous system structures by cancer cell |
Chemical substance | chemotherapy-induced neuropathy |
Metabolic diseases | diabetic neuropathy, uremic neuropathy |
Table 1: Factors triggering initiation of neuropathic pain.
Symptoms of neuropathic pain
Neuropathic pain is characterized by a set of symptoms, such as: spontaneous pain (stinging, burning, electrical shock-like), sensory impairments (paresthesia, dysesthesia, hyperalgesia, hyperpathy, allodynia) and vegetative symptoms being a consequence of damage to the nervous system structures caused by, among others, tumor tissue growth or cancer therapy (chemotherapy, radiotherapy, surgical procedures) (35). Action of various types of impulses/stimuli (chemical, mechanical, thermal) on nervous system tissue structures always triggers both functional and morphological changes, termed neuroplastic processes (19).
Neuropathic pain is characterized by a set of symptoms, such as: spontaneous pain (stinging, burning, electrical shock-like), sensory impairments (paresthesia, dysesthesia, hyperalgesia, hyperpathy, allodynia) and vegetative symptoms being a consequence of damage to the nervous system structures caused by, among others, tumor tissue growth or cancer therapy (chemotherapy, radiotherapy, surgical procedures) (35). Action of various types of impulses/stimuli (chemical, mechanical, thermal) on nervous system tissue structures always triggers both functional and morphological changes, termed neuroplastic processes (19).
Behavioral patterns described by animal owners that may indicate neuropathic pain include constant chewing, biting or scratching the same spot, spontaneous vocalization (whining/meowing) and defensive reaction to touch without any visible pathology. Hypersensitivity during examination of a suffering animal suggests neurological component of pain. To confirm it, the patient should be examined for hyperalgesia and allodynia (14, 28).
- Hyperalgesia occurs when an animal reacts defensively or aggressively to a painful stimulus (e.g. pin prick) applied either to the spot where the pain originates from (primary) or in surrounding areas (secondary).
- Allodynia (pain originating from intact tissues, not caused by any harmful non-painful stimulus – tactile allodynia) occurs when an animal reacts abnormally to an innoxious tactile stimulus in intact area, distant from primary hyperalgesia or hypersensitivity.
Neuropathic pain is initiated as a result of primary lesion or dysfunction in somatosensory part of the nervous system and persists for a long time, despite the cessation of the triggering factor. Unlike acute pain, it has no protective or warning function, often being not only a symptom of disease, but a disease process itself (3). The primary lesion can be caused by numerous agents (tab. I). Neuropathic pain can last for years, even decades after its initiation by triggering factor. This pain is described as stinging, tickling, "shooting", electrical shock-like, often of dysesthesia nature (unpleasant sensation arising spontaneously or under the influence of a stimulus). Neuroplastic processes, i.e. morphological and functional changes in the nervous system structures, take place in its course (19). Functional neuroplasticity leads to alterations at molecular and cellular levels. At the molecular level, transcription and/or translation is observed (38), evidenced by increased activity and number of ion channel receptors, decreased neuronal excitability threshold and facilitated spreading of specific molecules (e.g. endocytosis) and excitation in neuronal cells. At the cellular level, neuropathic pain leading to development of neuroplasticity initiates central sensitization resulting from – among others – sensitization of spinal dorsal horn neurons, which translates into lowering of pain threshold, increased response to stimuli and increased spontaneous neuronal activity caused by death of spinal dorsal horns cells (3).
As mentioned before, specific symptoms seen in patients suffering from neuropathic pain are hyperalgesia (abnormally increased sensitivity to pain) and allodynia (perceiving as noxious stimuli that are innoxious in healthy individuals, e.g. tactile stimuli) (35).
Neuroplastic processes cause functional disruption of nervous system and subsequently lead to the development of pain symptoms specific to neuropathic pain, including hyperalgesia and allodynia – sensory impairments resulting from formation of pain stimuli in response to both nociceptive and non-nociceptive stimulation (38, 42).
Neuropathic pain accompanies numerous diseases, such as postherpetic neuralgia, diabetic polyneuropathy (30), compressive neuropathy, multiple sclerosis or cancer and poses serious therapeutic problem (24). Medications currently used in neuropathic pain belong to antidepressants, anticonvulsants, opioid analgesics, selective serotonin reuptake inhibitors, local anesthetics (lidocaine, capsaicin) and NMDA receptor antagonists (33, 34, 40). Despite the undeniable progress over recent years in the treatment of this type of pain, currently available therapy is often insufficient. Patients suffering from neuropathic pain frequently exhibit resistance to analgesic drugs (especially opioid analgesics) and pharmacotherapeutics available for them often have serious side effects (33). For this reason, alternative solutions in the treatment of neuropathic pain are being intensively sought. High expectations for development of novel therapeutics are associated with metabotropic glutamate receptor (mGluR) ligands, particularly group I antagonists (mGluR1 and 5) (10, 11, 16).
Pathomechanisms of neuropathic pain
In theories explaining peripheral neuropathic pain formation, attention is drawn to instability of the nociceptors’ stimulation threshold and their high plasticity caused by peripheral sensitization. The development of hypersensitivity to pain is mediated by local release of chemical mediators termed „inflammatory cocktail” and accumulation of inflammatory reaction cells (17, 21). Mediators stimulate nociceptors directly (H+ ions, ATP, 5-HT) or indirectly, by sensitization to subsequent stimuli (bradykinin, SCFAs, PGF, NGF) (5, 13, 29). The next stage is the migration of inflammatory cells (macrophages, mastocytes, fibroblasts), which – by producing and releasing interleukin 1-β, tumor necrosis factor TNF-α and nerve growth factor NGF – contribute to decrease in nerve excitability threshold and production of spontaneous discharges in A-δ and C fibers (15, 39). Through activation of second messengers (cAMP, phosphokinase A and C), persistent inflammation elicits changes in gene transcription and posttranslation processes, which leads to disturbances in receptors’ structure and function (17). Significant role is played by formation of pathological connections, termed ephapses, between afferent nociceptive and sympathetic fibers, which explains development of pain related to stimulation of the sympathetic nervous system (complex regional pain syndrome) (41, 43). Similar phenomenon termed „sprouting” of nerve terminals from inner spinal dorsal horn laminae (III and IV) to II lamina has been described in A-β fibers. As a consequence, the input signal informing about mechanical stimuli is perceived as nociceptive (6). The process of peripheral sensitization also engages ion channels of nerve fibers, which include voltage gated ion channels: Na v1.3, Na v1.7, Na v1.8, Na v1.9, TRPV1. Their excessive expression at the site of nerve damage and in intact dorsal root ganglia (DRG) leads to ecotopic, spontaneous discharges with high frequency pulses (7). In recent years, high significance is attributed to changes in CNS as consequences of propagation of peripheral lesions.
Central mechanisms involve sensitization of spinal dorsal horn neurons, which results in lowering of the pain threshold, increased reactivity to stimuli, increased spontaneous activity (23) and ultimately death of spinal dorsal horn cells. The major role in these processes is played by excitatory amino acid – glutamate (Glu), ligand of AMPA receptors, whose rapid and repetitive stimulation causes formation of fast synaptic potentials and removal of Mg2+ ions blocking ion channel associated with to N-methyl-D-aspartate receptor (NMDAR). Activation of NMDA receptors leads to intracellular Ca2+ influx in neurons and their enhanced excitability. This results in activation of cyclooxygenase and lipoxygenase and consequent production of proinflammatory cytokines (8, 9, 17, 27). Sources of pathological pain are also sought at higher levels of the nervous system. Brainstem is the center of the modulating system consisting of inhibitory and excitatory descending pathways. Excitatory pathways, originating in periaqueductal grey matter (PAG) and rostral ventromedial medulla (RVM), are responsible for maintaining central sensitization. On the other hand, reduced activity of descending inhibitory pathways – where 5-HT and E are mediators – is observed in chronic inflammation, which explains the efficacy of antidepressant drugs blocking the uptake of these neurotransmitters in neuropathic pain. However, so far no one succeeded in convincingly explaining why the balance between these pathways is upset and why in some animal models of neuropathic pain activity of inhibitory pathways dominates, and in others the excitatory descending system is active (12, 22).
According to Wordliczek and Zaj?czkowska (38, 39), neuropathic pain, by causing neuroplastic processes, induces functional changes in the nervous system at cellular level, by triggering central sensitization, and at molecular level, i.e. modification of transcription and/or translation processes (increase in activity and number of receptors/ion channels, decrease in the neuronal excitability threshold, change in the location of individual molecules, e.g. endocytosis). Central sensitization is the effect of pathological, temporal and spatial postsynaptic summation, i.e. depolarization of larger number of neurons in spinal cord dorsal horns (SCDH) and extension of neuronal receptive fields, as a result of which subthreshold stimuli become suprathreshold. Clinically, changes at cellular level are manifested by long-lasting hyperalgesia, which persists even without nociceptive stimulation, still after the tissues or organs have healed. As a result of functional neuroplasticity, changes in the neural network occur, consisting of impulse generation and formation of calcium wave in neurons. Changes in postsynaptic mechanisms in SCDH neurons initiate positive feedback in the form of enhanced neuronal hypersensitivity, which further increases the wind-up mechanism of receptor activation, as well as the release of pronociceptive transmitters (39).
Structural neuroplasticity induces changes at synaptic level and in neuronal network. At synaptic level, enhancement of Rac1 protein activity (signaling protein regulating protein kinases activity, thereby increasing the density of synapses) and/or attenuation of Homer 1a protein activity occurs. Second of mentioned above proteins significantly inhibits NMDA and AMPA activation. Receptors’ inhibition results in suppression of Ca2+ ions release from sarcoplasmic reticulum, which inhibits MAP kinase activation (by, among others, reducing the number of synaptic connections).
At the level of neuronal network, structural neuroplasticity induces changes in the form of neurotrophic disorders leading to development of e.g. neuropathy or hyperplasia/sprouting of nerve fibers (figure 1), typical of patients suffering from bone pain due to tumor metastases to bones (38), in whose marrow very intense pain sensation develops. Neuropathic pain substantially alters the number of nervous cells. Microglia and astrocytes proliferation and degradation of inhibitory interneurons are observed.
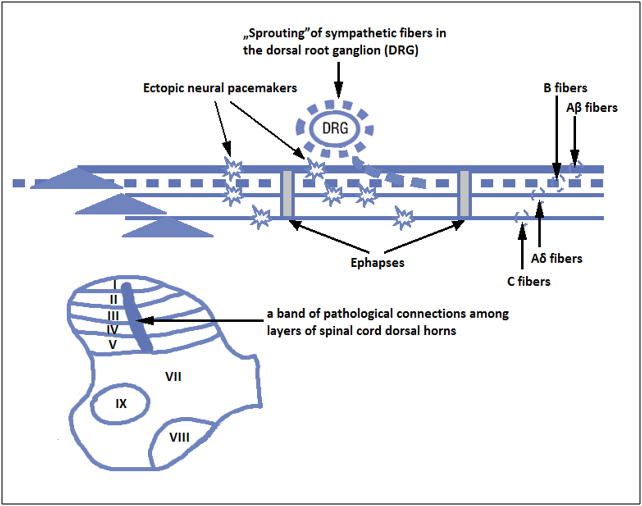
Picture 1: Diagram of the mechanism of pathological neuropathic pain (DRG – dorsal root ganglion) (courtesy of pos. 38).
Implications of pathological pain
Impulses received by nociceptors reach SCDH, where they are gated by enkephalinergic inhibitory interneurons, then pass to the higher CNS structures, in which they undergo a second gating (inhibition by endogenous anti-nociceptive systems) at the reticular formation level and ultimately reach medial prefrontal cortex, where they are processed. During development of chronic (pathological) pain, numerous diverse alterations occur in the nervous system. Those include:
Impulses received by nociceptors reach SCDH, where they are gated by enkephalinergic inhibitory interneurons, then pass to the higher CNS structures, in which they undergo a second gating (inhibition by endogenous anti-nociceptive systems) at the reticular formation level and ultimately reach medial prefrontal cortex, where they are processed. During development of chronic (pathological) pain, numerous diverse alterations occur in the nervous system. Those include:
- Internalization of GluA2 subunits in AMPA SCDH receptors, leading to significant acceleration of Ca2+ transport through ligand-gated ion channels coupled to AMPA.
- Selective degradation of GABAergic interneurons in SCDH after partial nerve lesion, resulting from increased glutamatergic stimulation, which in turn leads to activation of caspase and apoptosis. The consequence of these alterations is disruption of balance between excitation and inhibition in the nervous system.
- Disinhibition facilitating transmission of nociceptive signals through Aβ fibers by, among others, excitating interneurons stimulating PKCγ in lamina II of SCDH. After nerve damage, some of the Aβ fibers sprout to neurons that were disinhibited as a result of strong inhibition of GABAergic inhibitory effects.
- Increased activity of enzymes dependent on increased Ca2+ concentration (COX-2, NOS) in SCDH, leading to release of pronociceptive neurotransmitters, such as PGE2 or NO.
- Reduction in chloride gradient caused by nerve damage. This results in decreased activity of postsynaptic KCC2, i.e. cotransporter maintaining K+ - Cl- gradient, which in turn enhances postsynaptic neurons excitability. This process potentiates brain derivative neuronal factor (BDNF) released from microglia. Attenuation of cotransporter KCC2 activity is also associated with pathogenesis of chronic pain syndromes (spinal cord injuries, diabetic neuropathy), (38).
- Increased microglia activation after nerve injury caused by ATP and chemokine fractalkine release. Stimulation of Toll-like and purinergic receptors induces BDNF, which – by activating TrkB receptors in lamina I of SCDH – enhances neuronal excitability and pain response to both nociceptive and non-nociceptive stimulation (allodynia, hyperalgesia).
- Activation of kinases (ERK, CaMKIV) and cAMP affecting transcription of genes involved in formation of „pain memory” and induction of long-term changes in pain sensitivity by lowering the pain threshold.
- CREB activation in neuronal cell nuclei by cAMP and ERK, which induces expression of genes encoding nociception-related proteins (including COX-2 and TRPV1) and DREAM (transcription inhibitor that inhibits the expression of prodynorphin in SCDH) and increases nociception.
As a result of processes listed above, synthesis of novel protein molecules on RNA gene matrix and synthesis of new membrane receptors may occur. This situation alters neuronal activity for a longer period of time, i.e. several days, sometimes even permanently, causing development of chronic pain syndrome. Other changes occurring after nerve damage due to a disease process are presented on figure 1 (figure 1) and described in recently published articles (38, 39).
Glutamate receptors (iGluR and mGluR)
Glutamate (glutamic acid – Glu) is main excitatory neurotransmitter in the central and peripheral nervous system of vertebrates and plants, exerting its functions via ionotropic (iGluR, including AMPA, NMDA and kainate receptors) and metabotropic receptors (mGluR). mGluR receptors are classified into III groups, regarding their sequence homology, pharmacological profile and signal transduction mechanisms (table II).
Glutamate (glutamic acid – Glu) is main excitatory neurotransmitter in the central and peripheral nervous system of vertebrates and plants, exerting its functions via ionotropic (iGluR, including AMPA, NMDA and kainate receptors) and metabotropic receptors (mGluR). mGluR receptors are classified into III groups, regarding their sequence homology, pharmacological profile and signal transduction mechanisms (table II).
Group | Subtype | Localization | Class of G protein | Effect after stimulation |
I | mGluR1, 5 | predominantly postsynaptic | Gq /G11 | activation of the inositol phospholipid pathway |
II | mGluR2, 3 | predominantly presynaptic | Gi/o | cAMP inhibition |
III | mGluR4, 6, 7, 8 | predominantly presynaptic | Gi/o | cAMP inhibition |
Table 2: Types of metabotropic glutamate receptors.
Group I receptors (mGluR1 and mGluR5) are predominantly coupled to Gq/G11 protein (18). Their stimulation results in phospholipase C (PLC) activation and subsequent inositol trisphosphate (IP3) production, release of intracellular Ca2+ storages and protein kinase C (PKC) activation (31). Group I mGluRs are localized predominantly postsynaptically, where they are activated by high concentration of Glu in the synaptic cleft; however, there are also reports of their presynaptic location. On the contrary to group I, group II and III receptors are located predominantly presynaptically. Their stimulation elicits attenuation of adenylate cyclase (cAMP) activity and ultimately reduction of Glu release (37).
In recent years numerous evidences indicating a substantial role of mGluRs in the processes of neuropathic pain formation and modulation were provided (32). Up to now, most studies have concerned group I mGluR receptors, suggesting that their activation triggers spinal mechanisms mediating development of neuropathic pain symptoms. It was demonstrated that intrathecal administration of DHPG – a group I mGluR agonist – to rats results in development of thermal hyperalgesia and tactile allodynia typical for neuropathic pain. Numerous electrophysiological and behavioral studies provide evidence that group I mGluR agonists are able to enhance the SCDH neurons excitability and elicit increased sensitivity to mechanical and thermal stimuli, whereas antagonists reverse above actions, which was demonstrated using animal models of neuropathic pain. Studies using mGluR1 and mGluR5 antagonists will be discussed below (20, 36).
Conclusions
Neuropathic pain requires usage of several classes of drugs and procedures, due to the impossibility of managing it by utilizing single pharmacological or non-pharmacological method. Various analgesics and therapeutic methods are used before, during and after each surgery, aiming to reduce afferent stimulation of nociceptors. Administration of many of them is continued after surgery to reduce sensation from both the peripheral nervous system and the central nervous system.
Promising outcomes of numerous studies using mGluR1 and mGluR5 antagonists in neuropathic pain models show potential for development of novel type of drug effective in the treatment of neuropathy. Unfortunately, currently known compounds being antagonists of mGluRs are inherent to serious side effects, which prevents their use in further phases of clinical trials. Further studies aimed at identifying non-competitive antagonists of group I mGluRs with both sufficiently high receptor affinity and a wide therapeutic window are required.
References
- Baron R. (2006). Mechanisms of disease: neuropathic pain - a clinical perspective. Nat. Clin. Pract. Neurol. 2, 95-106.
- Battaglia A.A. (2016). An introduction to pain and its relation to nervous system disorders. 1st Ed. John Willey&Sons, Ltd.
- Bet A. (2012). Powtarzalno?? zjawiska sumowania czasowego w modelu bólu neuropatycznego u zdrowych ochotników. AM Pozna? ss. 7-10.
- B?czyk E., ?uczak J., Kotli?ska-Lemieszek A. (8/1999). Patomechanizm i leczenie bólu neuropatycznego. Nowa Medycyna-Ból i Opieka Paliatywna I
- Cheng H.Y., Pitcher G.M., Laviolette S.R. (2002). DREAM is a critical transcriptional repressor for pain modulation. Cell 108, 31–43.
- Chien S.Q., Li C., Li H., Xie W., Pablo C.S., Zhang J.M. (2005). Sympathetic fiber sprouting in chronically compressed dorsal root ganglia without peripheral axotomy. J. Neuropathic Pain Symptom Palliation 1, 19–23.
- Chung K., Lee B.H., Yoon Y.W., Chung J.M. (1996). Sympathetic sprouting in the dorsal root ganglia of the injured peripheral nerve in a rat neuropathic pain model. J. Comp. Neurol. 376, 241–251.
- Coull J.A., Beggs S., Boudreau D. i wsp. (2005). BDNF from microglia causes the shift in neuronal anion gradient underlying neuropathic pain. Nature 438: 1017–1021.
- Dib-Hajj S.D., Binshtok A.M., Cummins T.R., Jarvis M.F., Samad T., Zimmermann K. (2009). Voltage-gated sodium channels in pain states: role in pathophysiology and targets for treatment. Brain Res. Rev. 60, 65–83.
- Dogrul A., Ossipov MH., Josephine Laib J., Malan Jr.b TP., Porreca F. (2000). Peripheral and spinal antihyperalgesic activity of SIB-1757, a metabotropic glutamate receptor (mGLUR5) antagonist, in experimental neuropathic pain in rats. Neurosci. Lett. 292, 115-118.
- El-Kouhen S.G., Lehto J.B., Pan R., Chang S.J., Baker C., Zhong P.R., Hollingsworth J.P., Mikusa E.A., Cronin K.L., Chu S.P., McGaraughty M.E., Uchic L.N., Miller N.M., Rodell M., Patel P., Bhatia M., Mezler T., Kolasa G.Z., Zheng G.B., Fox A.O., Stewart M.W., Decker R.B., Moreland J.D., Brioni P., Honore P. (2006). Blockade of mGluR1 receptor results in analgesia and disruption of motor and cognitive performances: effects of A-841720, a novel non-competitive mGluR1 receptor antagonist. Brit. J. Pharmacol. 149, 761–774.
- Gao Y.J., Ji R.R. (2010). Chemokines, neuronal–glial interactions, and central processing of neuropathic pain. Pharmacol. Ther. 126, 56–68.
- Garry E.M., Moss A., Rosie R., Delaney A., Mitchell R., Fleetwood-Walker S.M. (2003). Specific involvement in neuropathic pain of AMPA receptors and adapter proteins for the GluR2 subunit. Mol. Cell. Neurosci. 24, 10–22.
- Harden, R. N. (2005). Chronic neuropathic pain. Mechanisms, diagnosis, and treatment. Neurologist 11, 11-22.
- Harriott B.S., Gold M.S. (2009). Contribution of primary afferent channels to neuropathic pain. Curr. Pain Headache Rep. 13, 197–207.
- Jaeschke G., Wettstein J.G., Nordquist R.E., Spooren W. (2008). mGlu5 receptor antagonists and their therapeutic potential. Expert Opin. Ther. Patents 18, 123-142.
- Jolivalt C.G., Lee C.A., Ramos K.M., Calcutt N.A. (2008). Allodynia and hyperalgesia in diabetic rats are mediated by GABA and depletion of spinal potassium-chloride co-transporters. Pain 140, 48–57.
- Kania B.F., Wro?ska D. (2018). Metabotropic glutamate receptors. Classification, structure and role in diseases. W: O’Keeffe J. (ed.): Metbaotropic Glutamate Receptors. NOVA, USA, ss. 1-79.
- Kania B.F., Wro?ska D. (2017). Introduction to neurolplasticity. JBBS 7, 42-49.
- Kozela E., Pilc A., Popik P. (2003). Inhibitory effects of MPEP, an mGluR5 antagonist, and memantine, an N-methyl-D-aspartate receptor antagonist, on morphine antinociceptive tolerance in mice. Psychopharmacol., 165, 245–251.
- Kuner R. (2010). Central mechanisms of pathological pain. Review Nature Medicine 16, 1258-1266.
- Leung L., Cahil C.M. (2010). TNF-α and neuropathic pain – review. J. Neuroinflammation 7, 1-11.
- Lu Y., Zheng J., Xiong L., Zimmermann M., Yang J. (2008). Spinal cord injury-induced attenuation of GABAergic inhibition in spinal dorsal horn circuits is associated with down-regulation of the chloride transporter KCC2 in rat. J. Physiol. (Lond.) 586, 5701–5715.
- Mathews K., Kronen P.W., Lascelles D., Nolan A., Robertson S., Steagall P. V., Wright B., Yamashita K. (2014). Guidelines for recognition, assessment and treatment of pain. J. Small Anim. Pract. 5, E10–E68.
- Mathews K. A.: Neuropathic pain. Vet. Clin. North Am. Small Anim. Pract. 2008, 38, 1365-1414.
- Merskey H., Bogduk N. (eds). (1994). Classification of chronic pain. Task Force on Taxonomy. Part III: Pain Terms. A Current List with Definitions and Notes on Usage. 2nd Ed. IASP Press, Seattle 209-214.
- Milligan E.D., Watkins L.R. (2009). Pathological and protective roles of glia in chronic pain. Nature Rev. Neurosci. 10, 23–36.
- Muir, W.W. III. Wiese, A. J., Wittum, T. E. (2004). Prevalence and characteristics of pain in dogs and cats examined as out patients at a veterinary teaching hospital. J. Am. Vet. Med. Assoc. 224, 1459-63.
- Nicotra L., Loram L.C., Watkins L.R., Hutchinson M.R. (2012). Toll-like receptors in chronic pain. Exp. Neurol. 234, 316–329.
- Niessen, S. J., Powney, S. & Guitian, J. (2010). Evaluation of a quality-of-life tool for cats with diabetes mellitus. J. Vet. Intern. Med. 24, 1098-1105.
- Niswender C.M., Conn J.P. (2010). Metabotropic glutamate receptors: physiology, pharmacology, and disease. Annu. Rev. Pharmacol. Toxicol. 50, 295–322.
- Osikowicz M., Mika J., Przewlocka B. (2013). The glutamatergic system as a target for neuropathic pain relief. Exp. Physiol. 98, 372–384.
- Szczudlik A., Dobrogowski J., Wordliczek J., St?pie? A., Krajnik M., Leppert W., Woro? J., Przeklasa-Muszy?ska A., Kocot-K?pska M., Zaj?czkowska R., Janecki M., Adamczyk A., Malec-Milewska M. (2014a). Rozpoznanie i leczenie bólu neuropatycznego: przegl?d pi?miennictwa i zalecenia Polskiego Towarzystwa Badania Bólu i Towarzystwa Neurologicznego – cz??? pierwsza. Ból 15, 8-18.
- Szczudlik A., Dobrogowski J., Wordliczek J., St?pie? A., Krajnik M., Leppert W., Woro? J., Przeklasa-Muszy?ska A., Kocot-K?pska M., Zaj?czkowska R., Janecki M., Adamczyk A., Malec-Milewska M. (2014b). Rozpoznanie i leczenie bólu neuropatycznego: przegl?d pi?miennictwa i zalecenia Polskiego Towarzystwa Badania Bólu i Towarzystwa Neurologicznego – cz??? druga. Ból 15, 8-21.
- Treede R.D., Jensen T.S., Campbell J.N. (2008). Neuropathic pain: redefinition and a grading system for clinical and research purposes. Neurology 70, 1630–1635.
- Varty G.B., Grilli M., Forlani A., Fredduzzi S., Grzelak M.E., Guthrie D.H., Hodgson R.A., Lu S.X., Nicolussi E., Pond A.J., Parker E.M., Hunter J.C., Higgins G.A., Reggiani A., Bertorelli R. (2005). The antinociceptive and anxiolytic-like effects of the metabotropic glutamate receptor 5 (mGluR5) antagonists, MPEP and MTEP, and the mGluR1 antagonist, LY456236, in rodents: a comparison of efficacy and side-effect profiles. Psychopharmacol., 179, 207–217.
- Wiero?ska J.M., Cie?lik P. (2017).Glutaminian i jego receptory, czyli o tym, jak mo?na uleczy? mózg. Wszech?wiat 118, 178-189.
- Wordliczek J., Zaj?czkowska R. (2014). Ból neuropatyczny — patomechanizm. Medycyna Paliatywna w Praktyce 8, 2: 61–65.
- Wordliczek J., Zaj?czkowska R. (2013). Mechanisms in cancer pain. W: Cancer pain. Hanna M., Zylicz B. (red.). Springer, London Heidelberg New York ss. 47–70.
- Wordliczek J., Zaj?czkowska R. , Dobrogowski J. (2011). Farmakologiczne leczenie bólu neuropatycznego. Polski Przegl?d Neurol. 7, 39–48.
- Xua Q., Yaksh T.L. (2011). A brief comparison of the pathophysiology of inflammatory versus neuropathic pain. Curr. Opin. Anaesthesiol. 24, 400–407.
- Zhou Q., Wang J., Zhang X., Zeng L., Wang L., Jiang W. (2013). Effect of metabotropic glutamate 5 receptor antagonists on morphine efficacy and tolerance in rats with neuropathic pain.Eur. J. Pharmacol. 71, 17-23.
- Zimmermann M. (2001). Pathobiology of neuropathic pain. Eur. J. Pharmacol. 19, 23-37.
- Kumar N., Laferriere A., Yu J.S.C., Poon T, and Coderre T.J. (2010). Metabotropic glutamate receptors (mGluRs) regulate noxious stimulus-induced glutamate release in spinal cord dorsal horn of rats with neuropathic and inflammatory pain. J Neurochem. July 114(1): 281–290.
Citation: Bogdan Feliks Kania and Urszula Bracha. (2020). The Neuropathic Pain in Animals. Archives of Veterinary and Animal Sciences 2(2). DOI: 10.5281/zenodo.3906136
Copyright: © 2020 Bogdan Feliks Kania. This is an open-access article distributed under the terms of the Creative Commons Attribution License, which permits unrestricted use, distribution, and reproduction in any medium, provided the original author and source are credited.