Research Article
Volume 2 Issue 1 - 2020
Micellar Copper Oxide and Evaluation of its Lubricating Properties
1Tribochemistry Consulting, Salt Lake City, Utah 84117, USA and University of Economy, Biotribology Laboratory, 85-229 Bydgoszcz, Poland
2Military University of Technology, Faculty of Mechanical Engineering, Warsaw, Poland
2Military University of Technology, Faculty of Mechanical Engineering, Warsaw, Poland
*Corresponding Author: Zenon Pawlak, Tribochemistry Consulting, Salt Lake City, Utah 84117, USA and University of Economy, Biotribology Laboratory, 85-229 Bydgoszcz, Poland.
Received: May 05, 2020; Published: May 14, 2020
Abstract
The purpose of this paper is to study effectiveness of micellar copper oxide as antiwear additive for lubricating oil. The transfer phenomenon was studied for (steel-bronze) and (steel-steel) tribopairs with micellar copper oxide additive in transformer oil. The mechanism of wear reduction by micellar copper oxide is based on the formation of metallic copper durable tribofilm on the rubbing steel surface during the tribochemical reaction: Cu2+ + 2e-tribo → Cu°. A tribofilm containing metallic copper is formed on worn steel surface. The copper tribofilm formation can provide reasonably very low wear and friction coefficient. The micellar additive demonstrates a significant potential for further application as effective additive in oil formulation.
Key words: Micellar additive copper oxide; Lubricating oil; Wear and friction; Friction coefficient; Transfer phenomenon
Introduction
The formation of the tribofilm of pure copper was treated originally as a formation of a very thin soft film during copper alloy contact with steel under condition of boundary lubrication and was named transfer phenomenon [1-3]. The formation of the copper tribofilm was reported to be followed by a very low friction coefficient < 0.01 and practically zero wear. Copper oil-soluble compounds, such as copper naphthalene and oleate, are good anti-oxidation additives [4] and micellar copper oxide can give maximum benefit when used as multi-functional additives in liquid lubricant, greases, fuels, cutting fluids, and hydraulic fluids [5-7]. A low friction coefficient (f = 0.003 - 0.004) occurs in lubricated sliding surfaces with copper and copper alloy coating made by electro-pulse spraying and its value is close to those in viscous media [8].
In recent years, attention has been shifted to the production and uses of nano-sized powders and liquid of MoS2, WS2, h-BN, and graphite, substantial reduction in friction and wear have been reported [9, 11]. However, copper additives should be considered in the first place because of their low cost, easy maintenance and effectiveness in lubricants [2, 11].
The combinations of (steel-bronze) and (steel-steel) tribopairs were studied in synthetic ester and mixtures with five different anti-wear additives. It was found that in boundary lubricated bronze–steel contact can give transfer of copper to steel a layer which gives a lower wear rate a friction coefficient [12]. Some engine oil additives, dissolved or dispersed in an oil formulation, exist in micellar forms as hard-core reverse micelles RMs or micelle aggregates [6, 11, 13].
The term reverse micelles (RMs) refers to the amorphous cores of cooper oxide solvated by surfactant molecules [3, 13]. The (Cumetallic) tribofilm formation requires that tribochemical reaction occurs between the reverse micelles (CuO)x(oleic acid)n and the activated metallic surfaces [6, 11, 14].
(CuO)x(oleic acid)n (RMs) + e-tribo → (Cumetallic)x – tribofilm (1)
The objective of this work is the evaluation of micellar copper oxide as antiwear additive for (steel-bronze) tribopair in oil using four-ball apparatus and friction tester. However, the effects of micellar copper on friction and wear properties of (steel-bronze) tribopair have not been fully established. Analyses of used oils for content of dissolved copper and iron were carried out to discuss triboreduction reaction 1). Also, other possible process should be considered:
2Fe+2 (or Fe°) + 3CuO + 2H2O → Fe2O3 + 3Cu° + 4H+ (2)
Materials and Methods or Experimental Procedures
Micellar copper oxide paste was prepared under conditions in which colloidal microparticles were formed according to chemical reaction (CuSO4 + NaOH) in the presence of oleic acid as the surfactant (CuO)x(oleic acid)n and subsequently dispersed in hydrocarbon oil. The concentration ratio of micellar microparticles to oleic acid and transformer oil were 2: 2: 1.5 by weight, respectively [9]. A micellar copper oxide paste was analyzed for content of heavy all metals and elemental analysis was reported [11]. The copper additive paste contained [mg/kg]: 108000 mg/kg of copper, 1780 mg/kg of nickel and about 100 mg/kg of arsenic, lead and iron. Metals concentration in micellar copper oxide additive and used oil samples were determined by inductive couple plasma-optical emission spectrometer Perkin-Elmer model 4300 DV. Digestion of samples of copper additive was carried out using EPA 3050B method [16].
The (CuO)x (oleic acid)n microparticles with size ranging between 30 to 40 µm in diameter were added as additive to transformer oil with different concentration (0.5 and 1.5 wt. %) and this oil was used as the base stock. The physical properties of transformer oil are: density at 20°C (g/cm3) 0.870 and kinematic viscosity (mm2/s) at 40°C 11.63 and at 60°C 3.4. The tested materials in this study were NC6 steel and B101 bronze. NC6 steel is a standard journal was composed [%]: C 1.3-1.45; Mn 04-07; P and S 0.03; Si 0.15-0.4; Cr 1.3-1.65; Ni 0.35; Mo 0.2; W 0.2; V 0.25; and Cu 0.35 and having its surface hardness of 62HRC, and the surface roughness up to Rz = 0.90 µm. B101 is a tin bronze (CuSn10P) and is composed [%]: Cu plus Sn 9-11, P 0.8-1.2.
Wear scar of various diameters were determined in the four-ball apparatus from Stanhope Seta Ltd (PN-C-04147). The operating conditions under which wear was measured were applied at load 32 N and temperature 25°C. The wear test was performed for transformer base oil and oil with (0.5 and 1.5 wt. %) of additive for periods of 5, 10, 15, 20 and 30 minutes (see Figure 2).
Friction test was carried out on friction tester apparatus (see Figure 1) [10]. The system characteristics of sliding bearing were determined with the (steel–bronze) bearing tribopair. All tests were performed at a sliding velocity 2.5 m/s, (1530 rpm) and duration of 140 minutes, Figure 3 and the dependence of friction on stress (load) duration time 200 minute, Figure 4. The dimension of the bronze bearing wasø25/ø36 x 20 (mm). Subsequent examination with NU2-Carl/Zeiss Jena microscope and micrometer showed that the steel metal and bronze bush surfaces were smooth.
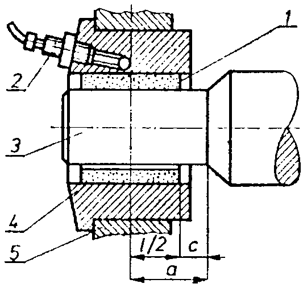
Figure 1: Schematic view of the friction tester apparatus showing the locations of its key components: bronze bearing (1), temperature-sensitive resistor (2), steel NC6 journal (3), clamping (4), rolling bearing (5).
Results and Discussion
The micellar copper oxide particles are easily dispersible in oils, fuel, and water forming a stable lubricant [7]. Transfer and adhesion of the micellar particles accelerate surface modification, self-reducing, and forming of a fine copper tribofilm. It is known that under boundary friction conditions triboemission can lead to the release of triboelectrons, charged particles, and photons [14]. Boundary friction and frictional energy induce the formation of a thin copper tribofilm. Since tribofilm formation takes place during the friction process, disintegration of the RMs occurs according to tribochemical reaction (see reaction 1).
Analysis of copper concentration in oil samples during the test in four-ball wear machine shows copper level has decreased from 216 mg/kg in fresh oil to 133, 85 and 43 mg/kg after run of 10, 30 and 60 min, respectively.
When formation of the copper layer on rubbed steel surface occurs (see reaction 1), some amount of iron is worn out from the surface as Fe metal particles or other Fe+2 species. This stays in oil formulation. Other processes for copper ion deposition in solution and on steel surface can be explained by contact substitution of iron ions by copper:
2 Fe+2 (or Fe°) + CuO + 2H2O → Fe2O3 + 3Cu° + 4H+ (2)
If sufficient amount of metallic iron is in contact with the copper micelles, iron dissolution (friction saturates the surface quicker) and copper deposition continue. The difference of redox potentials, ?E between the two redox couples is about 0.5 V: Fe3/Fe2 +0.80 V, and for Cu+2/Cu° is 0.3 V (in water scale) [7]. Redox potentials simply provide a quantitative measure of the case of oxidizing or reducing a given species in oil formulation.
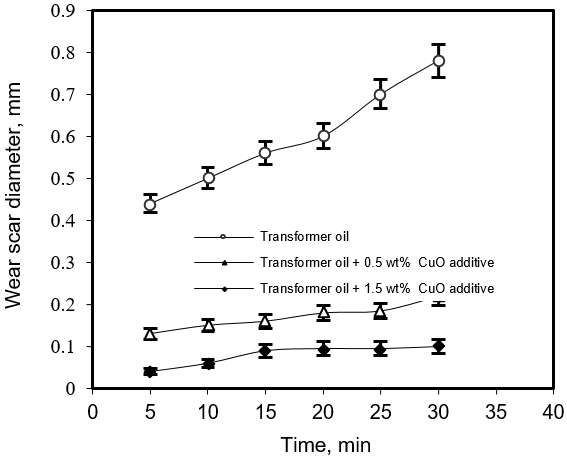
Figure 2: Wear scar diameter (WSD) vs. time for (steel/steel) tribopair in transformer oil and oil with 0.5 and 1.5 wt% CuO additive.
Anti-wear test conducted in the four ball machine under boundary conditions shown in Figure 2 resulted in WSD (wear scar diameter) values for transformer oil and oil containing 0.5 and 1.5% wt% CuO additive. It can be seen that the wear loss in WSD values with oil containing copper oxide additive are much lower than that of oil, which suggests that CuO additive has reduced wear very effectively.
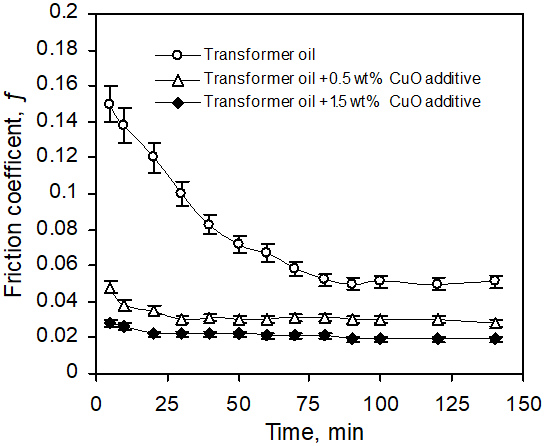
Figure 3: Friction coefficient (ƒ) vs. increasing duration time for (steel-bronze) tribopair in transformer oil, and transformer oil with (0.5 and 1.5) wt% CuO additive.
Figure 3 shows friction coefficient as function of increasing friction time in transformer oil and oil containing 0.5 and 1.5 wt% CuO additives. The main decrease of (ƒ) is observed in the initial sliding period, the material layer transferred from the bronze to the steel surface becomes continuous (Figure 3), reaching an optimum friction coefficient. It can be seen from the figure that the friction coefficient reached a steady state after showing a sharp decrease during the initial sliding period. The wear and friction in the experiment conditions, take place through materials transfer on (steel/bronze) couple lubricated with transformer oil and after 140 min a yellow-red colored thin porous layer was identified. It can be seen that the friction coefficient of the oil contains CuO additive is low and more stable than that of oil only. In the prolonged duration of friction tribopair (steel–bronze) in oil without additive, friction decreased from f = 0.15 to 0.06 after 1.2 hr operation and then stabilized.
Ilie investigated transfer phenomena on (steel-bronze) couples lubricated with glycerin [2] and demonstrated that the main decrease of the (ƒ) was done in 5-10 min, the covering surfaces with a porous red-yellow layer thickly 1-5 µm.
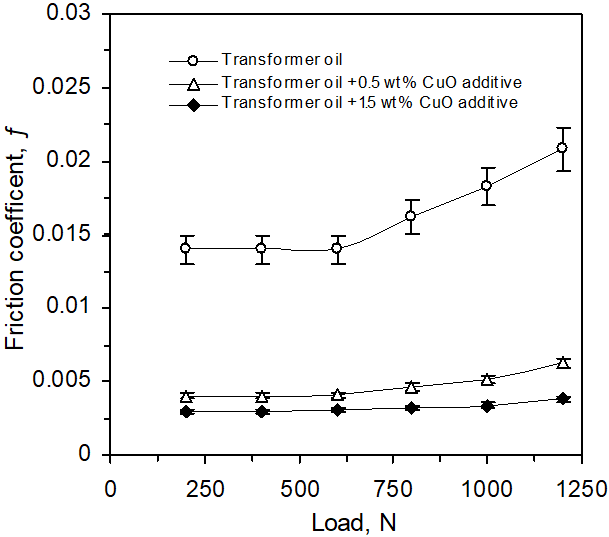
Figure 4: Friction coefficient (ƒ) vs. increasing normal load for (steel-bronze) tribopair in transformer oil, and transformer oil with (0.5 and 1.5) wt% CuO additive for sliding speed 2.5 m/s during 200 min run.
Figure 4 shows the friction coefficient as function of increasing applied load for transformer oil and oil containing 0.5 and 1.5 wt% CuO additive. The friction coefficient of lubricant with CuO additive is much lower than that with transformer oil only, indicating that CuO additive has good friction-reduction properties. It can be seen that the friction coefficient slowly increase with load increase until 600 N, then has a tendency of a sudden increase of (ƒ). Lubricant with CuO additive at different loads has a tendency of stabilization of (ƒ) around a low values.
During friction process Cu (II) is very reactive and easy adheres to steel surface. Literature data indicate that copper tribofilm is very thin [8] and low concentration of copper is needed to provide good lubrication. A small amount of soluble copper additive (less than 2000 ppm) dramatically improved oil quality. Greetham [17] reported that deposit was reduced from 6% to 0.5% and oil evaporation was reduced from 7% to none when oil with commercial additives was compared with oil containing copper additive.
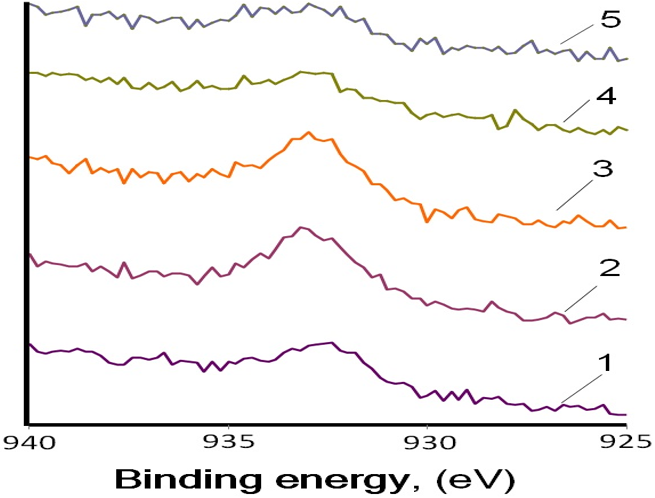
Figure 5: X-ray photoelectron spectroscopy (XPS) spectra of Cu2p: (curve 5) Background, (curve 4) Lithium grease LT34 under load 50N, (curve 3) CuO additive (grease) under load 5N, (curve 2) CuO additive (grease) under load 50N, (curve 1) Mineral oil SN650 +1.5 wt% CuO additive (grease) [18].
XPS analysis of the chemical states of some typical elements on the worn steel surfaces were performed, attempting to further confirmed the results of our tests the lubricating mechanism of (CuO additive grease, curve 2 and curve 3) and (oil + 1.5 wt% CuO, curve 1) additive used as a lubricant. Figure 5 shows XPS spectra of Cu2p: 932.6 eV on worn steel surface lubricated with CuO microparticles. Can be found that the relative intensity of elements Cu and Cu2O exist on the boundary film increased about 100% (curves 1, 2, 3) comparing with background (curve 4 and 5).
Conclusion
The results of wear four-ball (steel-steel) tribopair test showed that formation of Cu metallic tribofilm on rubbing surfaces reduces wear significantly. The copper-containing surface coating provides continued lubrication for ball bearing with less wear and lower friction. A tribofilm containing metallic copper was formed on worn steel surface. The copper tribofilm formation can provide reasonably very low wear and friction coefficient. The test data showed that the copper concentration was changing with running time, indicating chemical reaction with metal surface exposed through the wear process for steel-bronze tribopair. The results from this investigation show that CuO additives give thick tribofilms at both steel and bronze surfaces. For (bronze-steel) tribopair CuO additive give much lower wear than the base oil. In many formulations the copper is more effective than most traditional additives. Worn surfaces were examined using XPS to determine the friction mechanism.
References
- D.A. Rigney, (2000). Transfer, Mixing and Associated Chemical and Mechanical Processes the Sliding of Ductile Materials”, Wear, 245, 1-9.
- F. Ilie, (2006). “Studies and Researches Concerning the Tribological Behaviour of Friction Couple Functioning with Selective Transfer, Tribol. Intern. 39, 774-780.
- N.K. Myshkin, (2000). “Friction Transfer Film Formation in Boundary Lubrication”, Wear, 245, 116-124.
- M. Zhang, X. Wang, W. Liu, X. Fu, (2009). “Performance and anti-wear mechanism of Cu nanoparticles as lubricating oil additives”, Ind. Lub Tribol. 61, 311-318.
- D. Dowson, (1998). History of Tribology, 2nd edition, Professional Engineering Publishing, London
- Z. Pawlak, (2003). “Tribochemistry of Lubricating Oils”, Elsevier, Amsterdam.
- GP. Shpenkov, (1995). “Friction Surface Phenomena”, Elsevier, Amsterdam.
- J. Podgurskas, V. Snitka, V Jankauskas and A. Andriušis, (2000). “Selective Transfer Phenomenon in Lubricated Sliding Surfaces with Copper and Its Alloy Coatings Made by Electro-Pulse Spraying”, Wear,260, 652-661.
- Erdemir, (2005). “Review of Engineered Tribological Interfaces for Improved Boundary Lubrication”, Tribol. Intern. 38, 249-256.
- Z. Pawlak, T. Kaldonski, R. Pai, E. Bayraktar, A. Oloyede, (2009). “A Comparative Study on the Tribological Behaviour of the Hexagonal Boron Nitride (h-BN) as Lubricant Micro Particles - Additive in Porous Sliding Bearings for a Car Clutch”, Wear, 267, 1198-1202.
- Z. Pawlak, B.E. Klamecki, T. Rauckyte, GP, Shpenkov and A. Kopkowski, (2005). The Tribochemical and Micellar Aspect of Cutting Fluids”, Tribol. Intern. 38, 1-4.
- A. Pettersson, K, Elisabet and I. Minami, (2008). “Additives for Environmentally Adapted Lubricants - Tribo Film Formation”, Tribol. Online, 3, 168-172.
- J.L. Mansot, M. Hallouis and J-M. Martin, (1993). “Colloidal Antiwear Additives 2. Tribological Behavior of Colloidal Additives in Mild Wear Regime”, Colloids and Surfaces, A: Physicochem. Eng. Aspects, 75, 25-31.
- C. Kajdas, M.J. Furey, A.L. Ritter and G.J. Molina, (2002). “Triboemission as a Basic Part of the Boundary Friction Regime: A Review”, Lubr. Sci. 1, 223-254.
- S. Sagatowski, and G. Szpienkow, (1995). “Preparation of Additives for Lubricants, Cutting Fluids, and Fuels”, PL Patent 167710 B1.
- EPA Method 3050B, (1996). “Acid Digestion of Sediments, Sludges, and Soils”, Revision 2.
- G. Greetham, (1999). “Copper Applications in Automotive. Copper Racing to Success”. www. copper.org/innovation/1999/09/oil.html.
- K. Gocman, T.J. Kaldonski, T. Kaldonski and Z. Pawlak, An Evaluation Lubricating Properties of CuO and h-Boron Nitride (h-BN). Tribol. Online (In press).
Citation: Zenon Pawlak., et al. (2020). Micellar Copper Oxide and Evaluation of its Lubricating Properties. Archives of Chemistry and Chemical Engineering 2(1).
Copyright: © 2020 Zenon Pawlak. This is an open-access article distributed under the terms of the Creative Commons Attribution License, which permits unrestricted use, distribution, and reproduction in any medium, provided the original author and source are credited.