Review Article
Volume 2 Issue 1 - 2020
Drug Interaction with Botulinum toxin
Department of Aesthetic Orofacial, São Leopoldo Mandic Institute, Campinas, São Paulo, Brazil
*Corresponding Author: Elifas Levy Nunes, Department of Aesthetic Orofacial, São Leopoldo Mandic Institute, Campinas, São Paulo, Brazil.
Received: June 29, 2020; Published: July 08, 2020
Abstract
With the popularity of orofacial harmonization in different areas, health professionals must have a huge anatomical and physiological knowledge to avoid complications, and if it happens, know how to treat. The use of botulinum toxin is widely used worldwide, whether for aesthetic or functional purposes, but there is few researches in the literature covering (abrangendo) the real drug interaction that occurs with some medications when associated with botulinum toxin, being duty (sendo dever) of health professional to be extremely cautious in the anamnesis and provide these possible interactions to patients. In view of the fact, we gathered (reunimos) the main drug interactions explored in literature to assist the conduct in the treatment of professionals to avoid complications that can lead to death.
Keywords: Botulinum toxin; Drug; Interaction.
Abbreviations: BT: botulinum toxin; HF: haematoma frequency
Introduction
Clostridium botulinum produces 7 botulinum neurotoxin serotype (sorotipos) (A, B, C, D, E, F and G) with serology (sorológicas) differences. The toxin is formed by a heavy chain of 100kDa and a mild 50kDa, considering that the last one is responsible for the intracellular cleavage of proteins necessary for the transmission of acetylcholine by the neuromuscular junction. Some drugs that interact in the motor plate (in acetylcholine), can act at different times (synthesis, storage or release on its receptors). All subtypes help inhibit the release of acetylcholine at the neuromuscular junction of striated muscle fibers, causing a flaccid muscle paralysis. Although the neuromuscular junctions of the striated muscles are more sensitive to toxins, autonomous cholinergic nerves, responsible for sweat production and nocireceptors, are also affected (Azuley et al., 2016).
The neuromuscular junction has several preformed small vesicles containing acetylcholine (neutrotransmitter). While potential travels through the nerve and reaches its terminal, the vesicles join in the terminal membrane of the junction. The membranes merge, and acetylcholine is released into the synaptic cleft, which will bind to the post-synaptic muscle starting muscle contraction. What promotes their fusion and release is the synaptic fusion complex, formed by the group of proteins called SNARE (soluble Nethylmaleimidesensitive factor attachment protein receptors) (Azuley et al., 2016).
There are two types of SNARE proteins: one that contains acetylcholine and is present in vesicles (v-SNARE or SNARE VAMP-2 vesicle proteins), and another present in the neuronal membrane, formed by two proteins in the target membrane (t-SNARE). The union of v-SNARE, t-SNARE and another protein called MUNC18-1 forms the complex responsible for the fusion of the vesicle with the membrane, and by transmission of the neurotransmitter acetylcholine (this release occurs in less than 0.5ms by calcium influx) (Azuley et al., 2016).
If any change occurs in SNARE proteins, adequate fusion will not occur and acetylcholine will not be released. Exactly the point at which botulinum toxin acts in this SNARE complex. The light chain (cadeia) at the neuromuscular junction catalyzes the cleavage of SNARE proteins in the synaptic fusion complex. The light weight chain of toxins A,C and E catalyze the cleavage of snap-25 that is part of the complex. Type C also cleaves synthxine and types B,D,F and G catalyze a complex protein (synaptobrevin or VAMP). Regardless of the location of the cleavage, the fusion complex is deactivated, the vesicles don’t fuse (fundem) and no release of acetylcholine occurs, consequently, the muscle doesn’t contract (Azuley et al., 2016).
The use of muscle relaxants should be done with caution, recommending the reduction of the initial dose of the relaxant, or use drugs of intermediate action such as Vecuronium, instead of muscle relaxants that have a longer action (Sposito, 2004). Patil et al. (2016) and Li et al. (2005) through a literature review showed that there are no studies that actually prove the real drug interaction with the toxin, but caution should be exercised.
Neuromuscular blockers are substances that interrupt synaptic transmission of the myoneural plaque, as well as botulinum toxin. There are several types and classifications that interfere with the neurotrasmissor, or even, non-neuromuscular blocking substances interfere if administered in high doses. They are substances routinely used as adjuvants of general anesthetics to perform orotrothal intubation, facilitate mechanical ventilation and aid in neuromuscular relaxation during surgical procedures (i.e., laparoscopies and ophthalmologic surgeries).
Some classes of antibiotics interact with de botulinum toxin, being that the most have presynaptic action, decreasing the release of acetylcholine, but also has post-synaptic action. The one that is best know are aminoglycosides, polymyxins and lincosamides. The aminoglycosides are antimicrobials used to treat serious infections caused by gram-negative aerobic bacteria. They are nephrotoxic, ototoxic and act on neuromuscular blockade, and due to this disadvantages, the use has been questioned. The most common are: gentamicin, amikacin, spectinomycin and tobramycin. The neuromuscular block generated is rare and occurs by competition with the calcium channel, consequently decreasing the release of acetylcholine. Before that, if neuromuscular blockade occurs, the administration of calcium salts may reverse. The polymixins have a different mechanism of action from the others. Two polymixins are commercially available, colistin (Polymixin E) and polymixin B. Which inhibit the release of acetylcholine and reduce post-junctional sensitivity, even mechanism of action of botulinum toxin. And this block cannot be reversed with calcium (Azuley et al., 2016). Santos et al. (1981) did an animal research, the authors tested the hypothesis that aminoglycosides increase neuromuscular blockade associate botulinum toxin. The results showed that gentamicin and tobramycin increased neuromuscular blockade and could still led to the death some mices.
Believes that as soon as the drugs are eliminated by the body the symptoms decrease (Huang et al., 2000). Researches by L'Hommedieu et al. (1979) and Wang et al. (1984) showed that despite the different toxicities and speed of duration of the substances, they cause paralysis by similar mechanisms, through the blocking of the passage of stimulus through the neuromuscular junction, and that there is a synergism between the drug and botulinum toxin.
Acetylcholinesterase inhibitors act as toxin antagonists by increasing the amount of acetylcholine at the neuromuscular junction, re-establishing muscle contraction as shoots appear and acetylcholine release occurs again. The end of the action of acetylcholine occurs by enzymatic hydrolysis of the molecule, performed by acetylcholinesterase. Acetylcholinesterase inhibitors are: donepezila, galantamine, rivastigmine, edrophonium, neostigmine, pyridostigmine and physostigmine. They are commonly used in the treatment of myasthenia gravis and in anesthetic procedures (Azuley et al., 2016).
Succinylcholine provides skeletal muscle relaxation in surgeries or hospital procedures. It has rapid onset (início rápido) (30 seconds) but short action (5 to 10 minutes), due to hydrolysis by cholinesterases (Ex: butylcholinesterase in the blood). May cause neuromuscular blocking activity with aminoglycosides, parenteral and local anesthetics, capreomycin, citreated blood, clindamycin, lincomycin, polymyxins, cholinesterase inhibitors, exposure to neurotoxic insecticides, procainamide, and quinidine (Azuley et al., 2016).
Depolarizing blockers similar to curare: curare is a mixture of alkaloids found in South America used by Indians in hunting to immobilize and paralyze wild animals, with the most important component tubocurarine. When the curare join acetylcholine receptors, they can’t be activated, causing loss of muscle function and paralysis. Considering that the effects are reversible with anticholinesterase like (fisostigmine and neostigmine) and don’t cause damage to nerve fibers (Azuley et al., 2016).
Magnesium sulfate is used as an anticonvulsant that potenciates neuromuscular blockers, increasing their duration. Magnesium sulfate acts by decreasing the release of acetylcholine (pre-synaptic action), decreasing the excitability of the muscle membrane (post-synaptic action) and blocking the muscle's contractility me. As well as, the dose of any depolarizing blocker should be reduced in patients who have used magnesium sulfate (Azuley et al., 2016).
Aminoquinoline is used for malaria treatment. With the use of this drug, decrease the excitability of the motor terminal plate region, reducing responses to repeated nerve stimuli and acetylcholine. This drug can antagonize the actions of physostigmine on skeletal muscle equal to curare, which can lead to respiratory distress and dysphagia in patients with myasthenia gravis. Aminoquinoline intensifies the effect of neuromuscular blocking agents (such as botulinum toxin), potentiating the effect (Azuley et al., 2016). However, an in vitro study conducted by Solaja et al. (2008) tested the effects of chloroquine as antimalarial. One of its results showed that chloroquine is a potent inhibitor of the metalloprotease activity of botulinum toxin and can be used in the treatment of malaria or botulinum toxin overdose. Corroborating the study by Sheridan et al. (1996) who found that aminoquinolones antagonize the actions of the toxin. Simpson (1982) conducted an animal survey in rats showed that chloroquine and hydroxychloroquine were found as depressors of neuromuscular transmission. Consequently, muscle responses to potassium and cholinergic agonists decreased. Still in this context, the ability of chloroquine to antagonize botulinum toxin strongly suggests that the toxin is interanlized. As well as, in addition to chloroquine, short-chain amines alter the membrane penetration of various protein toxins, which antagonize the neuromuscular block actions of botulinum toxin.
Calcium channel blockers are antihypertensives that inhibit calcium channels, relaxing the smooth vascular muscles. They include verapamil, nifedipino, diltiazem and alodipino. When calcium entry into the cytoplasm initiates contraction of myocytes, with adverse effects in non-vascular smooth muscles (Azuley et al., 2016).
Cyclosporin are immunosuppressive agent related to interleukin-2 inhibition. In view of the adverse effects, it increases the neuromuscular blockade caused by vecuronium and atracurium (non-depolarizing neuromuscular blockers used in surgeries for muscle relaxation during anesthesia), enhancing the effect of the toxin (Azuley et al., 2016). According to Huang et al. (2000), the drug has been reported to cause neuromuscular blockage characterized by muscle weakness and ventilatory failure. The exact mechanism of action is unknown, but it is believed to be the result of anti-inflammatory or immunosuppressive effects on muscle or presynaptic calcium canal block.
Penicillamine is used for rheumatoid arthritis and Wilson's disease. It has a commercial name of Cuprimine, obtained by the degradation of penicillin, being an effective chelating agent of copper, mercury, zinc and lead. D-penicillamine binds to acetylcholine receptors and stimulates the synthesis of prostaglandin E-1, which occupies acetylcholine receptors, interfering with neuromuscular transmission promoting blockade (Azuley et al., 2016).
Oral anticoagulants, Ginko Biloba and Acetylsalicylic acid are substances that alter the coagulation system and may increase the risk of bruising or bruising at the site of application of the toxin (Azuley et al., 2016). Schrader et al. (2018) studied the haematoma frequency (HF) in patients with anticoagulation receiving botulinum toxin (BT) therapy. It was included 32 patients (16 females, 16 males, age 69.3 ± 10.0 years) with blepharospasm (n = 6), hemifacial spasm (n = 8), post-stroke spasticity (n = 16), and cervical dystonia (n = 2) received BT therapy whilst on anticoagulation (anticoagulation group, AG). 32 patients matched for disease, target muscles, age, and gender received identical BT therapy without anticoagulation (control group, CG). The International normalised ratio (INR) at the time of BT injection was in all patients within the recommended margins of 2.0 and 3.0 (mean 2.6 ± 0.27). The results are: HF was 3.0% in AG and 1.8% in CG (not significant). All hematomas occurred in blepharospasm patients (AG 5.2%, CG 2.6%, not significant) and hemifacial spasm patients (AG 3.9%, CG 2.9%, not significant), concluding that relevant haematomas do not occur, and, interruption of oral anticoagulation is not justified.
Vitamin B: There is a line of thought that vitamin B complex (B1, B6 and B12) influences the shorter duration of botulinum toxin, since it plays a key role in the regeneration of nerve endings and formation of new motor plates (Azuley et al., 2016). A study conducted by Tatlidede et al. (2012) aimed to verify if vitamin B accelerates muscle reconstruction in rats after injecting BT. Forty-four adult rats were selected divided into 3 groups, group 1 of which received only BT injection; group 2 received vitamin B before BT application; group 3 received vitamin B and BT together. During the research, the gastrocnemius muscle was evaluated before and 10 times after the application of BT. The results showed similar amplitudes, and it can be concluded that BT is not altered in the presence of vitamin B.
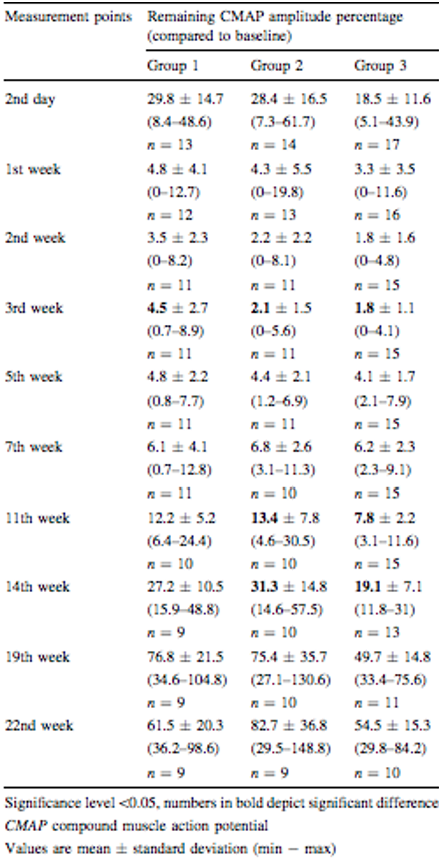
Source Tatlidede et al. (2012)
Table 1: Amplitude of the results obtained showing that there was no difference in the groups in relation to administration of Vitamin B and BT.
Table 1: Amplitude of the results obtained showing that there was no difference in the groups in relation to administration of Vitamin B and BT.
The morphine has been associated associated with botulinum toxin in treatment for chronic trigeminal pain with satisfactory results (Vlah et al., 2018). In addition, the study conducted by Vacca et al. (2012) tested the pharmacological interaction of BT with morphine in the fight against inflammatory pain, and morphine tolerance, in mices. A low BT dose of 2pg/paw was administered a day earlier associated with a low dose of morphine (1mg/kg) for 7 days, which generated significant analgesic action during the early and late stages. The results showed that BT can reduce the dose of morphine, preventing the development of tolerance to the drug. Besides that, Vacca et al. (2013) showed that the combinatory effects of BT and morphine could have an excellent therapeutic implication for sufferers of chronic pain.
DRUGS | INTERACTION |
ANTIBIOTICS | Potentiate BT |
ACETYLCHOLINESTERASE INHIBITORS | Decrease BT |
SUCCINYLCHOLINE | Potentiate BT |
CURARE | Potentiate BT |
MAGNESIUM SULFATE | Potentiate BT |
QUININE | Potentiate BT |
CALCIUM CHANNEL BLOCKERS | Mechanism not explained |
CYCLOSPORINE | Potentiate BT |
PANCURONIO, ROCURONIO, VERUCONIO and GALAMINE | Potentiate BT |
PENICILLAMINE | Potentiate BT |
Adapted of Toxina botulínica na Dermatologia. Source: Azulay et al. (2016)
Table 2: Summary of drugs interaction with botulinum toxin.
Table 2: Summary of drugs interaction with botulinum toxin.
Materials and Methods or Experimental Procedures
We used Pubmed, MEDLINE and Scielo using the keywords already cited issues from 1972 until 2018, about drug interaction and botulinum toxin. Some classes of drugs can interact physiological at the neuromuscular junction, increasing or decreasing the potential of botulinum toxin.
Results
Observing the previous researches, we could notice that most drugs potentiate the action of the toxin by acting in a similar way, decreasing the release of acetylcholine, with the exception of Acetylcholinesterase Inhibitors and Calcium Channel Blockers who don’t have relevant clinical research showing their real effect
Discussion
The results at this research, there are most important for us on day to day, health professionals, showed that in relation to antibiotics, more specifically aminoglycosides, competition occurs with the calcium channel, decreasing the release of acetylcholine, potentiating the action of BT. The Acetylcholinesterase inhibitors act as toxin antagonists because they increase the amount of acetylcholine at the neuromuscular junction, re-establishing muscle contraction. Magnesium sulfate potentiates neuromuscular blockers by increasing their duration because happens a decreasing the release of acetylcholine, blocking muscle contraction. Calcium channel blockers inhibit calcium channels by relaxing muscles, and consequently potentiating the effect of BT. Penicillamine binds to acetylcholine receptors, competing with BT and consequently decreasing its effect. (Azuley et al., 2016).
Solaja et al. (2008), Simpson (1982) and Sheridan et al. (1996) showed that aminoquinolines due to its potential for action, it can be used in the treatment of malaria or overdose of botulinum toxin. Corroborating the research of Huang et al. (2000) in which this drug antagonizes paralysis caused by BT, acting on the cell membrane inhibiting the binding or internalization of the toxin inside the cells.
Cyclosporine increases neuromuscular blockade by potentiating the effect of BT (Huang et al., 2000).
Oral anticoagulants, ginko biloba and acetylsalicylic acid alter the coagulation system, increasing the risk of bruising and bruising when associated with BT (Schrader et al., 2018).
Morphine is widely used in the treatment of chronic trigeminal pain, and has been questioned in relation to its interaction with BT. being associated with an increased analgesic effect and decreased tolerance to morphine (Vacca et al., 2012; Vlah et al., 2018).
Finally, many professionals believe that vitamin B influences the shorter duration of BT because it is associated with regeneration of nerve endings and formation of new motor plates (Azuley et al., 2016). But, a research by Tatlidede et al. (2012) with albino mices has shown that the use of vitamin B does not interfere with the efficacy of BT.
Conclusion
We could conclude with this literature review that some drugs compete in the neuromuscular junction acting on acetylcholine, in the same way as BT. In view of this fact, health professionals who perform this type of procedure should have extreme caution and knowledge about the interaction of drugs that potentiate or decrease the effects of the toxin.
Acknowledgments
Gather acknowledgements including the list of individuals who aided during the research at the end of the article in a separate section before the references.
Gather acknowledgements including the list of individuals who aided during the research at the end of the article in a separate section before the references.
References
- Azulay MM, Cavalcante R, Cirino P. (2016). Toxina Botulínica na Dermatolgia. 1ED: 136-141.
- Huang W, Foster JA, Rogachefsky AS. (2000). Pharmacology of botulinum toxin. J Am Acad Dermatol. 43(2 Pt 1): 249-259.
- L'Hommedieu C, Stough R, Brown L, Kettrick R, Polin R. (1979). Potentiation of neuromuscular weakness in infant botulism by aminoglycosides. J Pediatr.; 95(6): 1065-1070.
- Molgó J, Lemeignan M, Thesleff S. (1987). Aminoglycosides and 3,4-diaminopyridine on Neuromuscular Block Caused by Botulinum Type A ToxiN. Muscle Nerve 10(5): 464-470.
- Patil et al. (2016). Botulinum Toxin: Pharmacology and Therapeutic Roles in Pain States. Curr Pain Headache Rep; 20(3): 15.
- Pittinger C, Adamson R. (1972). Antibiotic blockade of neuromuscular function. Annu Ver Pharmacol 12: 169-184.
- Santos JI, Swensen P, Glasgow LA. (1981). Potentiation of Clostridium Botulinum Toxin Aminoglycoside Antibiotics: Clinical and Laboratory Observations. Pediatrics 68(1): 50-54.
- Schrader C, Ebke M, Saberi FA, Dressler D. (2018). Botulinum Toxin Therapy in Patients With Oral Anticoagulation: Is It Safe? J Neural Transm (Vienna).; 125(2): 173-176.
- Sheridan RE, Deshpande SS, Nicholson JD, Adler M. (1997). Structural features of aminoquinolines necessary for antagonist activity against botulinum neurotoxina. Vol 35: 9: 1439-1451.
- Simpson LL. (1982). The interaction between aminoquinolines and presynaptically acting neurotoxins. J of Pharmacology and Experimental Therapeutics (1) 43-48.
- Solaja et al. (2008). Novel 4-Aminoquinolines Active agains Chloroquine-Resistant and Sensitive P. falciparum Strains that also Inhibit Botulinum Serotype A. J Med Chem.; 51(15): 4388-4391.
- Souza et al. (2006). Influence of Nifedipine on the Neuromuscular Block Produced by Atracurium and Cistracurium. Study in Rat Phrenic-Diaphragmatic Nerve Preparation. Ver Bras de Anestesiol; 56: 2: 157-167.
- Sposito MMM. (2004). Toxina botulínica tipo A – propriedades farmacológicas e uso clínico. ACTA FISIÁTR. V.11 N.SUPL.1
- Tatlidede et al. (2012). Does Vitamin B Alter the Efficacy of Botulinum Toxin? Aesthetic Plast Surg.; 36(3): 692-697.
- Vacca V, Marinelli S, Eleuteri C, Luvisetto S, Pavone F. (2012). Botulinum neurotoxin A enhances the analgesic effects on inflammatory pain and antagonizes tolerance induced by morphine in mice. Brain Behav Immun.; 26(3): 489-499.
- Vacca V, Marinelli S, Luvisetto S, Pavone F. (2013). Botulinum toxin A increases analgesic effects of morphine, counters development of morphine tolerance and modulates glia activation and μ opioid receptor expression in neuropathic mice. Brain Behav Immun.; 32: 40-50.
- Vlah VD, Filipovic B, Bach-Rojecky L, Lackovic Z. (2018). Role of Central Versus Peripheral Opioid System in Antinociceptive and Anti-Inflammatory Effect of Botulinum Toxin Type A in Trigeminal Region. Eur J Pain;22(3): 583-591.
- Wang Y, Burr DH, Korthals GJ, Sugiyama H. (1984). Acute Toxicity of Aminoglycoside Antibiotics as an Aid in Detecting Botulism. Appl Environ Microbiol. Nov; 48(5): 951–955.
Citation: Carolina Guassi Mannina and Elifas Levy Nunes. (2020). Drug Interaction with Botulinum toxin. Journal of Oral Care and Dentistry 2(1). DOI: 10.5281/zenodo.3937965
Copyright: © 2020 Elifas Levy Nunes. This is an open-access article distributed under the terms of the Creative Commons Attribution License, which permits unrestricted use, distribution, and reproduction in any medium, provided the original author and source are credited.