Research Article
Volume 7 Issue 1 - 2025
Microplastic Distribution, Removal Techniques and Analysis: A Review
1Department of Chemistry, Ekiti State University, PMB 5363 Ado-Ekiti, Nigeria
2Department of Industrial Chemistry, Ekiti State University, PMB 5363 Ado-Ekiti, Nigeria
3Department of Chemistry and Biochemistry, Islamic University in Uganda, Mbale, Uganda
2Department of Industrial Chemistry, Ekiti State University, PMB 5363 Ado-Ekiti, Nigeria
3Department of Chemistry and Biochemistry, Islamic University in Uganda, Mbale, Uganda
*Corresponding Author: Olayinka Abidemi Ibigbami, Department of Chemistry, Ekiti State University, PMB 5363 Ado-Ekiti, Nigeria.
Received: January 08, 2025; Published: January 17, 2025
Abstract
The worldwide boost in plastic production has precipitated a manifold microplastic contamination problem. These minute plastic particles of size are ubiquitous in the environment and their effects on human beings are their result. Conversely, analysis of plastic particles in marine environment is a well-known, while that concerning microplastics within terrestrial ecosystems remains under explored. The present paper reviews the toxicological effects of microplastics on terrestrial ecosystems and human health and assesses the present and developing methods for their effective removal from the environment. In this paper, methodologies for the analysis of microplastics with an emphasis on distribution patterns, toxic effects, advanced analytical techniques, and new technologies for removal are reviewed. We also discuss specific sampling methods that differ from one environment to the other, noting some problems with each of them and detailing their limitations. We consider the possible risks arising from aging microplastic behaviour—bearing in mind its property evolution in time and impact. The prevailing study will add extensively to the improvement of microplastic analysis and removal techniques by providing insights and real practical recommendations that will help researchers and practitioners concerned with the problem at large.
Keywords: Microplastic; Persistent organic; Mass spectrophotometry; Removal technology
Introduction
Worldwide plastic manufacture surpassed virtually millions of metric tons per year. In conformity to the study conducted by Jambeck et al. (2015) which was issued in 2015, an estimated billion metric tons of concrete garbage was generated by 192 countries with a total population of over a billion people, an estimated million metric tons of it was plastic. Large plastic items dumped into nature over time decompose into small fragments referred to as microplastic (MPs). Microplastics are classified as tiny microfibers, whereas particles tinier than one micron are referred to as nanoplastics. According to Barnes et al., (2009) and Rocha-Santos et al. (2015), these particles are ubiquitous in the environment. There can be a differentiation of microplastics into two types: primary and secondary. Primary microplastics are generated to be microscopic in size or even less and can be found in personal care products, factory corrosives, and eventually imprint fabrics. On the other hand, secondary microplastics come from larger items that get degraded into smaller fragments by physical, chemical, and biological processes. Examples are foam from straws and agricultural mulching films. At present, increasing levels of microplastic pollution have come to be a major global concern, with several researches going on about their distribution, toxicity, analysis, and removal. Of the 192 countries around the world, 44 of these have conducted studies on microplastics. It has been noted that about 38% of the research is done on fish, while other organisms, like turtles, which are highly affected, have less than 1% attention to them in various studies. The regular plastics used for these purposes are polymers like polyester and polyamide. International plastic production consisted of significant proportions of polymers, and others, Plastics Europe, (2019). Among them, the major fractions of microplastics are polyester, and acrylic (Efimova et al., 2018; Jones et al., 2020). However, polyamide is commonly detected as the second primary microplastic on beaches (Efimova et al., 2018). The major sources of microplastics in freshwater include municipal and industrial wastewater, marine litter, and runoff water. Domestic sewage accounts for a good percentage of microplastic particles from personal care products, cleansers, and exfoliating beauty products that end up in the sewage during face washing and showering activities among others. Many countries use proficient water treatment methods to treat their sewage. While most microplastics in wastewater are removed through these sewage treatment plants, tiny plastic particles still persist in processed water (Carr et al., 2016; Sun et al., 2019). The problems linked to microplastics are considered here according to their distribution, toxicity, detection techniques and strategies for their removal. We would like to mention the circulation of microplastics in different environments and how they are consequently affecting ecosystems and human health. Current methodologies for detecting and quantifying microplastics were examined, focusing on their strengths and weaknesses. Moreover, this comprehensive review is directed to provide a background for exploring new removal strategies that have been tailored to mitigate microplastic presence in both water and soil, coupled with the discussion of the set of regulatory frameworks needed to drive effective mitigation of microplastic pollution.
Microplastic Distribution
Whereas establishing the actual magnitude of microplastic contamination is not easy, they have constantly been noticed in saltwater, freshwater, edibles, atmosphere, and soil. Several studies are projected to create an alarming future with further degrading conditions of microplastic pollution. Research estimates that between 1.15 and 2.41 million tons of plastic enter the world’s oceans via rivers annually, most of it derived from countries with rapidly developing economies but unsatisfactory waste management systems in Asia. The microplastic concentration might rise from moderate to high in the future, which is by far an increase in the concentration measurement, according to Kim (2020). Microplastics in the environment can originate from various pollution sources, and atmosphere deterioration, among others (Wanget al., 2020). This complex source generation of microplastics underlines the complexity of the measures to be adopted to solve the problem since they are related to different activities and processes. However, there is an easy transfer of lightweight microplastics by air, thus influencing terrestrial and marine environments.
Whereas establishing the actual magnitude of microplastic contamination is not easy, they have constantly been noticed in saltwater, freshwater, edibles, atmosphere, and soil. Several studies are projected to create an alarming future with further degrading conditions of microplastic pollution. Research estimates that between 1.15 and 2.41 million tons of plastic enter the world’s oceans via rivers annually, most of it derived from countries with rapidly developing economies but unsatisfactory waste management systems in Asia. The microplastic concentration might rise from moderate to high in the future, which is by far an increase in the concentration measurement, according to Kim (2020). Microplastics in the environment can originate from various pollution sources, and atmosphere deterioration, among others (Wanget al., 2020). This complex source generation of microplastics underlines the complexity of the measures to be adopted to solve the problem since they are related to different activities and processes. However, there is an easy transfer of lightweight microplastics by air, thus influencing terrestrial and marine environments.
Terrestrial microplastic distribution
The investigation into plastic particle pollution on the soil has become less advanced than that in the marine field of research. Studies on microplastics in terrestrial environments, whether soil or air, are tiniest with respect to the studies on seawater as well as sewage plant analysis. For instance, Tibbetts (2018) performed an assessment on microplastics in the sediments of River Tame and tributaries in Birmingham, where they found microplastics in all the sediment samples, with an average concentration. Mason (2018) determined that 93% of 259 bottled water samples from different locations and brands contained microplastic particles.Recent research increasingly identifies freshwater as a primary pathway and origin of microplastics in the environment. For instance, Ockelford (2020) mentioned that river networks, in particular during floods, contribute up to 80% of plastic entering the oceans. Galloway (2017) also pointed out that lots of plastic particles resolve in the environment which is the primary root of particles. This establishes a linkage between the pollution of freshwater and the ocean with microplastics; human activities like inhabitants' mass and economic development along rivers immensely influence marine microplastic contamination. Furthermore, it has been found that rainfall and flooding also play a very significant role in moving microplastics from land to water bodies. Huang et al. (2020) proved that sewage sludge usage and plastic coverage were major contributors to microplastics in soils. In contrast, Dioses-Salinas and Park (2020) found that the discharge of wastewater and contaminated irrigation led to theaccumulation of microplastics in soils. It has been confirmed by research that sludge actually carries more microplastics as compared to treated wastewater and hence serves as a major source of contamination in the soil when it is reused as a fertilizer. According to Corradini et al.(2019), microplastics dominate areas closely related to human activities, and their findings suggest that agricultural soils may be acting as important storage areas for microplastics due to the wide application of sludge-based fertilizers. Dioses-Salinas et al. (2020) have also researched the system of the origin of plastic particles, local topography, and hydrological properties in modulating microplastic concentrations in soils. In summary, research relating to microplastics in soils is becoming quite active, particularly in agricultural contexts. Since the application of wastewater treatment sludge contaminated with microplastics can disseminate into soils, monitoring sludge-based fertilizers should be an important part of strategies for controlling microplastic pollution on land. Moreover, as a solid particle medium in the environment, sediment has to be considered an important factor in soil-related microplastic studies since it is extracted, according to Harris et al. (2020), plastic particles should be taken as a kind of sediment due to their physical characteristics.
The investigation into plastic particle pollution on the soil has become less advanced than that in the marine field of research. Studies on microplastics in terrestrial environments, whether soil or air, are tiniest with respect to the studies on seawater as well as sewage plant analysis. For instance, Tibbetts (2018) performed an assessment on microplastics in the sediments of River Tame and tributaries in Birmingham, where they found microplastics in all the sediment samples, with an average concentration. Mason (2018) determined that 93% of 259 bottled water samples from different locations and brands contained microplastic particles.Recent research increasingly identifies freshwater as a primary pathway and origin of microplastics in the environment. For instance, Ockelford (2020) mentioned that river networks, in particular during floods, contribute up to 80% of plastic entering the oceans. Galloway (2017) also pointed out that lots of plastic particles resolve in the environment which is the primary root of particles. This establishes a linkage between the pollution of freshwater and the ocean with microplastics; human activities like inhabitants' mass and economic development along rivers immensely influence marine microplastic contamination. Furthermore, it has been found that rainfall and flooding also play a very significant role in moving microplastics from land to water bodies. Huang et al. (2020) proved that sewage sludge usage and plastic coverage were major contributors to microplastics in soils. In contrast, Dioses-Salinas and Park (2020) found that the discharge of wastewater and contaminated irrigation led to theaccumulation of microplastics in soils. It has been confirmed by research that sludge actually carries more microplastics as compared to treated wastewater and hence serves as a major source of contamination in the soil when it is reused as a fertilizer. According to Corradini et al.(2019), microplastics dominate areas closely related to human activities, and their findings suggest that agricultural soils may be acting as important storage areas for microplastics due to the wide application of sludge-based fertilizers. Dioses-Salinas et al. (2020) have also researched the system of the origin of plastic particles, local topography, and hydrological properties in modulating microplastic concentrations in soils. In summary, research relating to microplastics in soils is becoming quite active, particularly in agricultural contexts. Since the application of wastewater treatment sludge contaminated with microplastics can disseminate into soils, monitoring sludge-based fertilizers should be an important part of strategies for controlling microplastic pollution on land. Moreover, as a solid particle medium in the environment, sediment has to be considered an important factor in soil-related microplastic studies since it is extracted, according to Harris et al. (2020), plastic particles should be taken as a kind of sediment due to their physical characteristics.
Airborne microplastic distribution
Freshwater acts as a major transporter of microplastics to the ocean, but the air is by comparison a relatively understudied pathway compared to water and sediment. Because they are so light, however, microplastics can travel far on air currents and reach a wide range of environments both on land and at sea. Yukioka et al.(2020) analyzed the occurrence and characteristics of plastic particles in street dust from three locations. The researchers indicated that microplastic content in road dust varied between low and high stretches, and characteristics were extremely dissimilar among sites. In particular, an increased amount of elastic-derived microfibers has been found in road dust compared with dust from the general environment. This may indicate that sources like rubber and cable coatings might contribute to the microplastics present in highways. This discovery is in accord with Vogelsang et al. (2018), who assessed that tire scrape and road markings paint are the primary origins of microplastics in highways. Although, Yukioka et al. (2020) made these findings, they did not provide information on the precise occurrence and dispersal of microplastics in road dust. It stood rather difficult to trace specific sources. Further studies are therefore required to understand the distribution of microplastics in the atmosphere for estimating the levels of pollution by region or environmental conditions. A further study, Liu et al. (2019) examined microplastics in both indoor and outdoor across various Chinese cities and evaluated the day-to-day vulnerability of human beings to microplastics resulting from indoor dust. Their results showed indoor dust concentrations variedwidely, while outdoor dust concentrations were inconsistent. It has been affirmed that air is among the pathways through which microplastics move and spread, many more studies are required to identify clear sources, migration routes, distribution patterns, and toxicity of these microplastics to humans. Microplastic contamination cuts across all areas of the environment: water, land, and air.
Freshwater acts as a major transporter of microplastics to the ocean, but the air is by comparison a relatively understudied pathway compared to water and sediment. Because they are so light, however, microplastics can travel far on air currents and reach a wide range of environments both on land and at sea. Yukioka et al.(2020) analyzed the occurrence and characteristics of plastic particles in street dust from three locations. The researchers indicated that microplastic content in road dust varied between low and high stretches, and characteristics were extremely dissimilar among sites. In particular, an increased amount of elastic-derived microfibers has been found in road dust compared with dust from the general environment. This may indicate that sources like rubber and cable coatings might contribute to the microplastics present in highways. This discovery is in accord with Vogelsang et al. (2018), who assessed that tire scrape and road markings paint are the primary origins of microplastics in highways. Although, Yukioka et al. (2020) made these findings, they did not provide information on the precise occurrence and dispersal of microplastics in road dust. It stood rather difficult to trace specific sources. Further studies are therefore required to understand the distribution of microplastics in the atmosphere for estimating the levels of pollution by region or environmental conditions. A further study, Liu et al. (2019) examined microplastics in both indoor and outdoor across various Chinese cities and evaluated the day-to-day vulnerability of human beings to microplastics resulting from indoor dust. Their results showed indoor dust concentrations variedwidely, while outdoor dust concentrations were inconsistent. It has been affirmed that air is among the pathways through which microplastics move and spread, many more studies are required to identify clear sources, migration routes, distribution patterns, and toxicity of these microplastics to humans. Microplastic contamination cuts across all areas of the environment: water, land, and air.
Marine microplastic dispersion
Research into microplastics first focused on ocean contamination, dating back to 2014. Asindicated, a half-closed nature—in the case of seas, including the Mediterranean—results in an increased potential for the appearance of zones with concentrated contamination. Since then, several studies have been conducted related to microplastic presence in ocean waters, with special accentuation placed on surface layers. Iosobe et al.(2015) examined microplastics and mesoplastics in the Pacific off Japan and determined an absolute high concentration, which is far above the global ocean standard. In this regard, Russell and Webster (2021) sampled Scottish seas from 2014 to 2020, where the microplastic concentration ranged from varied lengths; in addition, fragments in 50% of the analysis existed as microfibers. Nonetheless, as a result of environmental and time-related differences in findings, no obvious movements of microplastic concentration were identified, as stated by Russell et al.(2021). Similarly, Pan et al. (2018) conducted a study where microplastics from notable sites in the Pacific, revealing the average concentration to be 10,000 MPs/km², while polyethylene makes up the major constituent as found by Pan et al. (2019).
Research into microplastics first focused on ocean contamination, dating back to 2014. Asindicated, a half-closed nature—in the case of seas, including the Mediterranean—results in an increased potential for the appearance of zones with concentrated contamination. Since then, several studies have been conducted related to microplastic presence in ocean waters, with special accentuation placed on surface layers. Iosobe et al.(2015) examined microplastics and mesoplastics in the Pacific off Japan and determined an absolute high concentration, which is far above the global ocean standard. In this regard, Russell and Webster (2021) sampled Scottish seas from 2014 to 2020, where the microplastic concentration ranged from varied lengths; in addition, fragments in 50% of the analysis existed as microfibers. Nonetheless, as a result of environmental and time-related differences in findings, no obvious movements of microplastic concentration were identified, as stated by Russell et al.(2021). Similarly, Pan et al. (2018) conducted a study where microplastics from notable sites in the Pacific, revealing the average concentration to be 10,000 MPs/km², while polyethylene makes up the major constituent as found by Pan et al. (2019).
Lindeque et al. (2020) suggested that this might result in the minimization of the abundance of microfibers. Using these nets, the same area was checked for the concentration of microplastics with 100, 333, and 500 μm, and they proved that the 100 μm mesh captured 2.5 to 10 times more microplastics than bigger meshes. The categories, sources and potential mitigation of upstream microplastic pollution is presented in Table 1.
Category | Source | Potential Mitigation |
Production | Microplastics in additives | Removing them from products. Replace with benign alternatives |
Mismanaged preproduction pellets | Regulate pellet handling. Operation clean sweep | |
Commerce | Industrial abrasives | Improve containment and recovery and require alternatives |
Laundromat exhaust | Improved filtration | |
Agriculture-degraded film, pots, and pipes | Improve recovery, biodegradable plastics | |
Consumer | Tire dust | Technological advances, road surface |
Littering of small plastic items (cigarette filters, torn packaging corners, small film wrappers, etc.) | Enforcement of fines for littering, Consumer education, EPR on design |
|
Domestic laundry. Wastewater effluent | Wash with top-load machines, wastewater containment, single-filter woven textiles, textile coatings | |
Waste management | Fragmentation by vehicles driving over unrecovered waste | Improved waste management |
UV and chemically degraded terrestrial plastic waste | Improved waste management | |
Sewage effluent (synthetic fibers) | Laundry filtration, textile industry innovation | |
Combined sewage overflow (large items) | Infrastructure improvement | |
Mechanical shredding of roadside waste during regular cutting of vegetation (mostly grass) | Better legislation and law enforcement; valorization of waste products |
Source: Eriksen et al. (2018)
Table 1: Categories, Sources and potential mitigation for upstream microplastic pollution.
Table 1: Categories, Sources and potential mitigation for upstream microplastic pollution.
Investigation associated with the operation of deep-water seaside plant life environments in cutting off microplastics is still in an emerging phase. Jones et al. (2020) explored the descent conduct of plastic particles in wetlands and subsea regions amidst sea grasses, which was the first of its kind to detect microplastics in the Zostera marina. Piarulli et al. (2020) did research on salt marshes and proved that 96% of the 330 samples analyzed had no traces of microplastics. Variations were noted in size, shape, and type of polymer ingested microplastics, as documented by Piarulli et al. (2020). Cozzolino et al. (2020) conducted a study on microplastic contamination of vegetation zones within the Ria Formosa lagoon, Portugal. The outcomes suggested that the restraint of plastic particles into sediment was elevated in subtidal zones than in intertidal areas, although they did not seem to be an effect from the vegetation on these concentrations.
However, scientific research so far is still limited because all these are being carried out on certain vegetation types only, making it hard to understand the extent of microplastic contamination across different vegetation zones. With expanding studies on microplastics in the aquatic habitat, there is a growing need for studies considering climatic and spatial variability of microplastic occurrence, distribution, and characteristics.
Microplastic Toxicity
While microplastics themselves represent an environmental hazard, when organic contaminants bind to these minuscule particles, they can give rise to even more serious problems. Recently, studies regarding microplastic toxicity have begun taking into consideration the combined effects of microplastics and other substances. For example, Zhou et al. (2020) found that mixing microplastics with PPCPs could lead to toxicity in organisms. It has been shown that microplastics can serve as vectors, carrying such toxicants to facilitate their dissemination. Not all studies show an increase in toxicity, however. Mixing microplastics with copper, Weber et al. (2021) did not find the effect of increased toxicity in experiments with water snails, Lymnaea stagnalis, for example. A further study by Gao et al.(2019) evaluates the interactions of microfibers with heavy metals, including lead, copper, and cadmium, in the marine environment. The authors established that some types of microplastics, such as polyvinyl chloride and polypropylene, showed a greater tendency to absorb those metals compared to others, such as polyamides, polyethylene, and polyformaldehyde (Gao et al., 2019). Meanwhile, testing carried out by Xu et al.(2021) exposed zebrafish (Danio rerio) to microplastics; no lethal effects were observed, but they reported higher concentrations of microplastics related to changes in survival, body length, and heartbeat in fish.
While microplastics themselves represent an environmental hazard, when organic contaminants bind to these minuscule particles, they can give rise to even more serious problems. Recently, studies regarding microplastic toxicity have begun taking into consideration the combined effects of microplastics and other substances. For example, Zhou et al. (2020) found that mixing microplastics with PPCPs could lead to toxicity in organisms. It has been shown that microplastics can serve as vectors, carrying such toxicants to facilitate their dissemination. Not all studies show an increase in toxicity, however. Mixing microplastics with copper, Weber et al. (2021) did not find the effect of increased toxicity in experiments with water snails, Lymnaea stagnalis, for example. A further study by Gao et al.(2019) evaluates the interactions of microfibers with heavy metals, including lead, copper, and cadmium, in the marine environment. The authors established that some types of microplastics, such as polyvinyl chloride and polypropylene, showed a greater tendency to absorb those metals compared to others, such as polyamides, polyethylene, and polyformaldehyde (Gao et al., 2019). Meanwhile, testing carried out by Xu et al.(2021) exposed zebrafish (Danio rerio) to microplastics; no lethal effects were observed, but they reported higher concentrations of microplastics related to changes in survival, body length, and heartbeat in fish.
Microplastics add to the accumulation of cadmium in the organs, and fish gills hence leading to cellular stress and immune response (Stock et al., 2019). What has been deduced is that generally, smaller microplastics cause more harmful effects over time—particularly on algal growth—in the cases where experiments combining the toxicity of various materials have been conducted. Conducted experiments both on living rats and using humans cell cultures (Stock et al., 2019). The authors reported a reactive oxygen species response in male mice, showing the impact on abdominal immune tissues, although no tissue damage was perceived. Cell experiments proved the possibility of tiny plastic particles entering cells, but on the whole, this study concluded that there were no serious health problems resulting from ingesting microplastics in mammals. Equally, the detection on how microplastics damaged the human stomach was studied, and it was determined that nanoscale microplastics may possibly intensify cell toxicity (Wang et al., 2020). Pop et al.(2021) studied the impact of bisphenol A on microorganisms in water and established that BPA had a likelihood of being an agent able to compromise cell membrane integrity and survival, thus posing risks to chlorophyll in these organisms. According to Bhatnagar and Anastopoulos (2017), cosmetics normally contain BPA, which is a known endocrine disruptor. Besides, it is found in surface water, groundwater, and wastewater. Figure 1 showed the schematic diagram of microplastic and nanoplastic toxicity in humans.
Wang et al. (2017) mentioned that phthalates directly impacted the endocrine system, leading to damage to the reproductive organs, especially during pregnancy and in the stage of childhood growth. Due to limited research on microplastic assimilation in human beings, the results should be interpreted carefully. Some recent studies have been performed regarding the environmental risk of microplastics on ecosystems. Thus, the calculated PNEC for freshwater species; the result showed that in parts of Asia, an ecological risk from microplastics cannot be excluded. Besseling et al. (2019) reviewed the toxicity data of 66 studies and estimated the risk of microplastic pollution to sensitive species in certain hotspot areas. They assessed the characteristics of microplastics at numerous sampling locations. Although risk assessment on microplastics in both humans and the environment is already in flow, more research is required to establish clear standards for microplastic concentration and to enhance the accuracy of risk assessment.
Microplastic Analysis
There is a dire need to develop scientific methods for the quantification and differentiation of microplastics; otherwise, a proper understanding of their environmental impacts will not be realized. Measurement and analytical techniques for microplastics as technologies are not yet well developed. They are plagued by an intrinsic insufficiency of detection sensitivity and speedin processing. Hence, scientists pay much attention to the accuracy and efficiency of microplastic analysis techniques. Such would be the general key stages for the analysis of microplastics in water: sampling, followed by pretreatment methods, and lastly by analytical methods.
There is a dire need to develop scientific methods for the quantification and differentiation of microplastics; otherwise, a proper understanding of their environmental impacts will not be realized. Measurement and analytical techniques for microplastics as technologies are not yet well developed. They are plagued by an intrinsic insufficiency of detection sensitivity and speedin processing. Hence, scientists pay much attention to the accuracy and efficiency of microplastic analysis techniques. Such would be the general key stages for the analysis of microplastics in water: sampling, followed by pretreatment methods, and lastly by analytical methods.
Sampling methods
Different methods of sampling microplastics exist when the target is water, sediments, soil, cosmetics, and living organisms. This review, therefore, limits itself to the most commonly applied methods in sampling within water and sediments.
Different methods of sampling microplastics exist when the target is water, sediments, soil, cosmetics, and living organisms. This review, therefore, limits itself to the most commonly applied methods in sampling within water and sediments.
Water sampling
The choice of the method of sampling microplastics in the water column depends on factors of its density, shape, properties, flow of the water, and depth at which water is taken for sampling (Campanale et al., 2020). Because the buoyancy of microplastics is influenced by water salinity, which further varies with the location and depth of water, this needs to be understood before thecollection of any samples. Two typical methods for the sampling of water are net sampling and bulk sampling. Some sampling devices used for the collection of MPs in surface waters were presented in Table 2.
The choice of the method of sampling microplastics in the water column depends on factors of its density, shape, properties, flow of the water, and depth at which water is taken for sampling (Campanale et al., 2020). Because the buoyancy of microplastics is influenced by water salinity, which further varies with the location and depth of water, this needs to be understood before thecollection of any samples. Two typical methods for the sampling of water are net sampling and bulk sampling. Some sampling devices used for the collection of MPs in surface waters were presented in Table 2.
Sampling device | Advantages | Disadvantages | Time (Minutes) | Reference |
Manta net | Sampling of large volumes of water; The lateral wings allow the floating of the device and the sampling of the water surface |
Expensive equipment; Requires boat; The lower limit of detection is 333 μm; Clogging problems; Risk of sample contamination; Underestimation of the total buoyant microplastic amounts. |
15–240 | Kang et al. (2015); Lenaker et al. (2019); Cutroneo et al. (2020) |
tNeuston net | Sampling of large volumes of water; Widely used (useful for comparing positions). |
Expensive equipment; Requires a boat; The lower limit of detection is 333 μm; Clogging problems; Risk of sample contamination; Underestimation of the total buoyant microplastic amounts. |
30 | McCormick et al. (2016); Syakti et al. (2018); Cutroneo et al. (2020) |
Plankton net | The lower limit of detection is 100 μm; Sampling of medium volumes of water; Possibility to sample the water column |
Expensive equipment; Requires a boat; Clogging problems; Sampling of lower volumes of water compared to Manta trawl; Risk of sample contamination; Underestimation of the total buoyant microplastic amounts |
30 | Dris et al. (2015); Amélineau et al. (2016); Cutroneo et al. (2020) |
Table 2: Sampling devices used for the collection of MPs in surface waters.
Source: Campanale et al. (2020)
Source: Campanale et al. (2020)
The common nets are surface trawl, paired plankton nets, and microfiber traps (Barrows et al., 2017; Campanale et al., 2020). The bulk sampling technique involves the filtering of a set volume of water through a container (Barrows et al., 2017). Net sampling allows the covering of huge areas and volumes, particularly from a boat (Abeynayaka and Cutroneo, 2020). However, the mesh size limits the size of the microplastic collected according to its mesh size (Von Ammon et al., 2020). For instance, manta trawls normally have a 330 μm mesh and hence large microplastics above this range are excluded from this type of collection mesh (Barrows et al., 2017). Nylon nets with a 100 μm mesh can be more effective in retaining microplastics, but fast clogging can happen with plankton and slow speeds are promoted in the collection (Abeynayaka et al., 2020). The recommended time for which the sampling is to be done is only one minute to ensure a low flow rate (Dris et al., 2015). Bulk or grab sampling collects a fixed volume of water but usually from a much smaller area than net sampling. In this possible alternative, technology has been developed to facilitate a microplastics sampler using a ship's pump, to allow sampling at high vessel speeds and instant size separation (SubCtech, 2020). Differences in these methods can result in different collections of the volume of microplastics (Campanale et al., 2020). Thus, net sampling is best used for larger areas of coverage with 100-350 μm net sizings based on microplastic size, and grab or bulk sampling is for smaller, confined areas.
Residue selection
Plastic particle dispersion in the residue is not even and may differ significantly during a collection based on a selection area, quantity of samples taken, and extraction depth. For example, residue collected from areas that are potential microplastic accumulation hotspots, such as tidal lines, will indicate a higher concentration. Shallower samples contain more microplastics, while the deeper samples (deeper than 15 cm) contain less microplastics (Willis et al., 2017). Sediment samples should be collected using a standard method of soil sampling. Soil samples are usually collected from the topsoil layer, which is 0-15 cm deep. Typically five to ten particles are selected either in an irregular format or from one center point and four other collection points around it. Afterward, these samples are combined and analyzed. The weight or volume speaks to the issue of representativeness, for which 300- 500g of the sample is recommended. The samples collected are put in glass bottles, chilled at 0 to 4 degrees, and dispatched to a lab. Upon arrival at the laboratory, the particles are dehydrated inside a furnace at 40°C for 72 hours, then moisture is measured. When dealing with a soil sample, Thomas et al. (2020) suggested sieving through meshes of sizes 1, 2, and 5 mm, which is within the normal available sieve sizes on the market.
Plastic particle dispersion in the residue is not even and may differ significantly during a collection based on a selection area, quantity of samples taken, and extraction depth. For example, residue collected from areas that are potential microplastic accumulation hotspots, such as tidal lines, will indicate a higher concentration. Shallower samples contain more microplastics, while the deeper samples (deeper than 15 cm) contain less microplastics (Willis et al., 2017). Sediment samples should be collected using a standard method of soil sampling. Soil samples are usually collected from the topsoil layer, which is 0-15 cm deep. Typically five to ten particles are selected either in an irregular format or from one center point and four other collection points around it. Afterward, these samples are combined and analyzed. The weight or volume speaks to the issue of representativeness, for which 300- 500g of the sample is recommended. The samples collected are put in glass bottles, chilled at 0 to 4 degrees, and dispatched to a lab. Upon arrival at the laboratory, the particles are dehydrated inside a furnace at 40°C for 72 hours, then moisture is measured. When dealing with a soil sample, Thomas et al. (2020) suggested sieving through meshes of sizes 1, 2, and 5 mm, which is within the normal available sieve sizes on the market.
Preliminary treatment technique
Assembled specimens consist of plastic particles mixed with dust, soil, and microbes. Such specimens require pretreatment to remove contaminants other than plastics. The general two steps in pretreatment are mass detachment and decomposition. For mass detachment, the normally used chemicals are NaCl, separating microplastics based on density from other materials. Oxidation involves substances like H2O2 for the elimination of natural organic matter.
Assembled specimens consist of plastic particles mixed with dust, soil, and microbes. Such specimens require pretreatment to remove contaminants other than plastics. The general two steps in pretreatment are mass detachment and decomposition. For mass detachment, the normally used chemicals are NaCl, separating microplastics based on density from other materials. Oxidation involves substances like H2O2 for the elimination of natural organic matter.
The order of these processes may vary depending on the sample and extent of contamination. In highly contaminated samples, pretreatment should be carried out to avoid interferences in inorganic particle measures before analysis. Because plastic particles and inorganic particles are tiny pieces that cannot be separated by visual separation, the differences in their densities are used to separate them. For performing this separation, various chemicals have been used as additives, like NaCl, NaBr, NaI, CaCl2 and ZnCl2. The common solution is the use of 1.2 kg/L the NaCl, 1.4–1.8 kg/L of sodium polytungstate, and 1.6–1.8 kg/L of NaI. Density separation can, however, be ineffective, especially for microplastics smaller than 1 mm, which have recovery rates as low as 40%, and more recently improved to 96–99%. Methods like centrifugation and air bubbling have been tested to decrease processing time. Nevertheless, there is no standardized method for the separation of microplastic density. Methods are then used to remove NOM from the surface of microplastics, such as 30% hydrogen peroxide and Fenton oxidation with different types of acid and base decomposition, after the separation of microplastics from inorganic particles. Although hydrogen peroxide removes organic matter efficiently, on their own they are unsuitable for plastics, particularly polyethylene and polypropylene, which usually need some combination of hydrogen peroxide and acids. As observed by (Al-Azzawi et al., 2020; Lusher et al., 2020; Thomas et al., 2020), H2O2 efficiently removes organic matter—sludge: 80–86%, soil: 96–108%. Still, it degrades the polymer. These acid treatments, however, can considerably destroy microplastics because of the low pH. NaOH digestion extensively dissipates PET and PC particles while effectively removing organic matter by 61–67% in sludge and 64–68% in soil. On the other hand, digestion with KOH had little impact on microfibers but effectively eliminated organic matter by 57% in sludge and 35% in soil (Al-Azzawi et al., 2020).
Analytical methods
A simple method for analyzing microplastics would be staining the microplastics with Nile Red dye, then allowing them to incubate for 10 minutes, followed by counting using a program (Kaile et al., 2020). This method is sometimes unreliable because the dye does not color all types of microplastics uniformly and includes non-plastic materials, hence bringing down the accuracy of the method (Kang et al., 2020). For example, the accuracy of the Nile Red staining technique is about 78% compared to the FTIR analytical method. While it may work in some microplastics under controlled conditions—for example, in distinguishing polypropylene particles in sand—it struggles to analyze more complex samples. Another technique uses UV-Vis measurements according to Beer's Law for the estimation of the number of microplastics, but it is only quantitative and hence subject to failure in case of particle movement or settling during analysis.
A simple method for analyzing microplastics would be staining the microplastics with Nile Red dye, then allowing them to incubate for 10 minutes, followed by counting using a program (Kaile et al., 2020). This method is sometimes unreliable because the dye does not color all types of microplastics uniformly and includes non-plastic materials, hence bringing down the accuracy of the method (Kang et al., 2020). For example, the accuracy of the Nile Red staining technique is about 78% compared to the FTIR analytical method. While it may work in some microplastics under controlled conditions—for example, in distinguishing polypropylene particles in sand—it struggles to analyze more complex samples. Another technique uses UV-Vis measurements according to Beer's Law for the estimation of the number of microplastics, but it is only quantitative and hence subject to failure in case of particle movement or settling during analysis.
Techniques so far can be separated into two primary categories: non-destructive ones, for instance, microscopy combined with FTIR or Raman spectroscopy, and destructive techniques like chromatographic methods coupled with spectrometric analysis. The commonly employed methods among them are FTIR or Raman microscopy and Pyrolysis-GC/MS (Muller et al., 2017; Wang et al., 2017).
Even though there is no single procedure accepted by all, the integration of tools such as CCD cameras, photodiodes, dynamic light scattering, and traditional methods put into pace rapid analysis techniques. For instance, researchers have measured the type and size of microplastics such as PET and LDPE using these tools. Still, the most used ones are FTIR microscopy and Pyr or TD/GC/MS, while frequent ones are LC/MS/UV and the Nile Red method. Results from FTIR and Nile Red methods are mostly given as several microplastics per liter or cubic meter, while or GC/MS and LC/MS, these are mostly weight per liter or cubic meter (Asamoah et al., 2019).
Methodology | Advantages | Disadvantages | Lower Size Limit | References |
Fourier transform infrared coupled to microscopy (μ-FTIR) | Easy to use Many particles can be analysed simultaneously Automatisation available Short time of analysis for single particles Evaluation of size and shape Detecting the intensity of oxidation Report particles with shape and size information Transmission and reflection mode Nondestructive Less expensive than Raman and thermoanalytical techniques |
Difficulty in characterising black particles Long time of analysis to measure multiple particles Measures huge areas without particles Detectors have to be cooled with liquid nitrogen The analysis requires expert personnel Huge data sets (several GB per filter) No total mass determination Expensive |
~10–20 μm | Käppler et al. (2016); Campanale et al.(2020); Primpke et al. (2020) |
Raman spectroscopy | Evaluation of size and shape Many particles can be analysed simultaneously Automatisation available Detecting the intensity of oxidation Staining possible Thermoelectrically cooled (TEC) detectors obviate the necessity for liquid nitrogen cooling Report particles with shape and size information Nondestructive Filter contributions can be subtracted out It is possible to detect additives, pigments, and plasticisers |
More time-consuming measurements with respect to FTIR-spectroscopy The analysis requires expert personnel Interference of biological and inorganic contaminants No total mass determination Expensive |
~1 μm | Käppler et al. (2016); Renner et al. (2018); Xu et al. (2019) |
Pyrolysis–Gas Chromatography-Mass spectrometry (Py–GC–MS) | More holistic approach to characterise, in a single analysis, additives and plasticiser, in addition to polymer category Powerful for mass determination |
No particle number information No evaluation of size and shape Particles can be analysed singularly About 40 min of analysis for each particle determination The analysis requires expert personnel Destructive Expensive |
~50/100 μm | Dümichen et al. (2015); Hendrickson et al. (2018); Funck et al. (2020) |
Table 3: Methodologies used to characterize MPs: advantages and disadvantages for each technique are described.
Source: Campanale et al. (2020)
Source: Campanale et al. (2020)
FTIR analytical technique
Measurement by Fourier Transform Infrared Spectroscopy serves as a noninvasive technique to analyze some polar functional groups—for example, carbonyl groups—based on the scattering of infrared light at specific wavelengths by the substance in question. Traditionally, FTIR has been unable to handle microplastics smaller than 500 μm and contaminated samples. However, micro(μ)-FTIR microscopy currently allows for the analysis of miniature plastic particles. The technique efficiently measures the entire regularity of thermal radiation in a single measurement through pretreated filter paper. Despite the effectiveness of these new techniques, FTIR spectroscopy remains limited. The techniques to enumerate small, irregularly shaped microplastics are time-consuming, and applying this method to samples of less than 20 μm remains quite challenging up to now (Tagg et al., 2015; Peihl et al., 2018).
Measurement by Fourier Transform Infrared Spectroscopy serves as a noninvasive technique to analyze some polar functional groups—for example, carbonyl groups—based on the scattering of infrared light at specific wavelengths by the substance in question. Traditionally, FTIR has been unable to handle microplastics smaller than 500 μm and contaminated samples. However, micro(μ)-FTIR microscopy currently allows for the analysis of miniature plastic particles. The technique efficiently measures the entire regularity of thermal radiation in a single measurement through pretreated filter paper. Despite the effectiveness of these new techniques, FTIR spectroscopy remains limited. The techniques to enumerate small, irregularly shaped microplastics are time-consuming, and applying this method to samples of less than 20 μm remains quite challenging up to now (Tagg et al., 2015; Peihl et al., 2018).
Raman analytical technique
Raman scattering shall be used for the identification of plastics by seeing how light scatters when molecules within particles of microplastic dimension get excited into various states, such as vibrational or rotational. Micro-Raman spectroscopy, in this case, is particularly applicable because it is capable of analyzing quite large areas of high resolution on microplastics and gives excellent selectivity and reproducibility. Besides, it only consumes a very small quantity of the sample with minimal preparation and fast data acquisition time cited in. However, this technique may be less efficient if the sample contains organic matter, which may produce unwanted fluorescence that hampers the analysis. Primpke et al. (2020) utilized spectroscopic analysis in analyzing seawater and reported that Raman detected almost 23% more microplastics than FTIR, mainly for particles ≤500 μm. It is in this respect that a newly developed Laser Direct Infrared chemical imaging system improves time analysis and resolution, providing key tool options to get ahead of both FTIR and Raman limitations which were dragging the process (Scircle et al., 2020).
Raman scattering shall be used for the identification of plastics by seeing how light scatters when molecules within particles of microplastic dimension get excited into various states, such as vibrational or rotational. Micro-Raman spectroscopy, in this case, is particularly applicable because it is capable of analyzing quite large areas of high resolution on microplastics and gives excellent selectivity and reproducibility. Besides, it only consumes a very small quantity of the sample with minimal preparation and fast data acquisition time cited in. However, this technique may be less efficient if the sample contains organic matter, which may produce unwanted fluorescence that hampers the analysis. Primpke et al. (2020) utilized spectroscopic analysis in analyzing seawater and reported that Raman detected almost 23% more microplastics than FTIR, mainly for particles ≤500 μm. It is in this respect that a newly developed Laser Direct Infrared chemical imaging system improves time analysis and resolution, providing key tool options to get ahead of both FTIR and Raman limitations which were dragging the process (Scircle et al., 2020).
Gas chromatography-mass spectrometry (GC/MS)
Gas chromatography-mass spectrometry involves heating a sample of microplastics and analyzing the produced gasses. Two key modes of Gas chromatography-mass spectrometry with respect to microplastic research: pyrolysis GC/MS and thermal desorption GC/MS. Both measure the ions developed during heating and thus give information on the amount of microplastics present. One of the strong points concerning GC/MS employment, in comparison with techniques such as FTIR and Raman spectroscopy, is its ability to realize both qualitative and quantitative analyses on very small samples, even for microplastics below 10 μg, due to the high sensitivity. One of the major disadvantages, however, is that GC/MS is destructive; during analysis, the sample is completely consumed, and thus there are no possibilities for further tests on the same particle (Bratton et al., 2018). Compared with Raman and FTIR techniques, which investigate the superficial nature of a microplastic particle and are subject to interventions by substances such as dyes, GC/MS is capable of examining the bulk of the particle and therefore represents a more complete analysis.
Gas chromatography-mass spectrometry involves heating a sample of microplastics and analyzing the produced gasses. Two key modes of Gas chromatography-mass spectrometry with respect to microplastic research: pyrolysis GC/MS and thermal desorption GC/MS. Both measure the ions developed during heating and thus give information on the amount of microplastics present. One of the strong points concerning GC/MS employment, in comparison with techniques such as FTIR and Raman spectroscopy, is its ability to realize both qualitative and quantitative analyses on very small samples, even for microplastics below 10 μg, due to the high sensitivity. One of the major disadvantages, however, is that GC/MS is destructive; during analysis, the sample is completely consumed, and thus there are no possibilities for further tests on the same particle (Bratton et al., 2018). Compared with Raman and FTIR techniques, which investigate the superficial nature of a microplastic particle and are subject to interventions by substances such as dyes, GC/MS is capable of examining the bulk of the particle and therefore represents a more complete analysis.
Microplastic Removal Techniques
Sewage removal technique
Coming from factory, household, farming, and animal wastewaters, there can be huge contributions of microplastics, although sewage remedy techniques are not competent to capturehem totally. Examples include wastewater containing micro pellets coming from some cosmetic products like scrubs or textile fibers released by washing. Eventually, when this wastewater reaches municipal treatment plants, large plastics are filtered out, but microplastic and nanoplastic particles persist through multiple remedy methods, so certain plastic particles continue to be released into the treated sewage. In this respect, the high residual of microplastics in sewage brings into focus the need for improving their removal efficiency. Accordingly, comprehensive research has been conducted. Preliminary screening and sedimentation are done as the initiation steps in treatment, mainly for the elimination of large plastic wastes. Primary treatment shall include aeration, then sedimentation, skimming off lighter plastics, or settling heavier ones. This is followed by secondary treatment focusing on biological processes, in which organic material is to be removed through a number of anaerobic, anoxic, and aerobic tanks, then settling tanks, which may effectively remove most microplastics over five hundred microns (Conley et al., 2019; Edo and Wu, 2020). Tertiary treatment in wastewater treatment plants, though mostly an optional process, uses chemical processes to remove phosphorus and nitrogen, and thus has the potential to enhance microplastic removal with the addition of coagulants.
Sewage removal technique
Coming from factory, household, farming, and animal wastewaters, there can be huge contributions of microplastics, although sewage remedy techniques are not competent to capturehem totally. Examples include wastewater containing micro pellets coming from some cosmetic products like scrubs or textile fibers released by washing. Eventually, when this wastewater reaches municipal treatment plants, large plastics are filtered out, but microplastic and nanoplastic particles persist through multiple remedy methods, so certain plastic particles continue to be released into the treated sewage. In this respect, the high residual of microplastics in sewage brings into focus the need for improving their removal efficiency. Accordingly, comprehensive research has been conducted. Preliminary screening and sedimentation are done as the initiation steps in treatment, mainly for the elimination of large plastic wastes. Primary treatment shall include aeration, then sedimentation, skimming off lighter plastics, or settling heavier ones. This is followed by secondary treatment focusing on biological processes, in which organic material is to be removed through a number of anaerobic, anoxic, and aerobic tanks, then settling tanks, which may effectively remove most microplastics over five hundred microns (Conley et al., 2019; Edo and Wu, 2020). Tertiary treatment in wastewater treatment plants, though mostly an optional process, uses chemical processes to remove phosphorus and nitrogen, and thus has the potential to enhance microplastic removal with the addition of coagulants.
The concentration and size of microplastics in influent wastewater to effluent vary according to factors such as the plant's location and wastewater characteristics, alongside treated objectives; further research is hence necessary.
Physical removal technique
Common mechanical techniques applied to microplastic removal include buoyancy separation, settling, and screening, of which the last one is the most broadly utilized. Filtration techniques incorporate screening, disk filters, and sand filters, while membrane types also use microfiltration, ultrafiltration, nanofiltration, dynamic membranes, and reverse osmosis. Screening is first of all used at most sewage remedy techniques to filter out bigger plastic particles through filtering and sedimentation. Research has shown that this screening technique is effective in removing 40%-80% of microplastics. Membrane filtration, in particular, is efficient, with a removal efficiency of over 90%, especially for microplastics larger than 10 μm. This topic has been explored in several studies (Kunz et al., 2016; Xu et al., 2019; Hebner et al., 2020; Kaile et al., 2020; Kang et al., 2020). However, the accumulation of microplastics on membranes accelerates contamination of the membranes themselves and traps other organic materials. Therefore, a pretreatment process involving membrane filtration is very essential to avoid over-contamination from microplastics and organic matter.
Common mechanical techniques applied to microplastic removal include buoyancy separation, settling, and screening, of which the last one is the most broadly utilized. Filtration techniques incorporate screening, disk filters, and sand filters, while membrane types also use microfiltration, ultrafiltration, nanofiltration, dynamic membranes, and reverse osmosis. Screening is first of all used at most sewage remedy techniques to filter out bigger plastic particles through filtering and sedimentation. Research has shown that this screening technique is effective in removing 40%-80% of microplastics. Membrane filtration, in particular, is efficient, with a removal efficiency of over 90%, especially for microplastics larger than 10 μm. This topic has been explored in several studies (Kunz et al., 2016; Xu et al., 2019; Hebner et al., 2020; Kaile et al., 2020; Kang et al., 2020). However, the accumulation of microplastics on membranes accelerates contamination of the membranes themselves and traps other organic materials. Therefore, a pretreatment process involving membrane filtration is very essential to avoid over-contamination from microplastics and organic matter.
Chemical removal technique
The chemical methods developed for the removal of microplastics have been implemented in many ways, among which coagulation and precipitation are the most used techniques for water treatment. In this respect, the efficiency of microplastic removal is highly dependent on the coagulant type, dosage, and coagulation time. Hence, research in this area remains continuous to determine the type of coagulant and its conditions that will ensure maximum removal. For instance, the research of Lapointe et al. (2020) conducted a Jar test to compare various microplastic removal rates with aluminum-based coagulants and polyacrylamide dosing on polyester and polyethylene microplastics. It points out the potential of chemical treatment, but many more studies are required to fine-tune these methods to gain even better results. Based on its review, PAM combined with aluminum- or iron-based coagulants is capable of removing as high as 99% of microplastics, depending on size, quantity, and aquatic parameters. The study also established that electrocoagulation can be an effective method for eliminating plastic particles.
The chemical methods developed for the removal of microplastics have been implemented in many ways, among which coagulation and precipitation are the most used techniques for water treatment. In this respect, the efficiency of microplastic removal is highly dependent on the coagulant type, dosage, and coagulation time. Hence, research in this area remains continuous to determine the type of coagulant and its conditions that will ensure maximum removal. For instance, the research of Lapointe et al. (2020) conducted a Jar test to compare various microplastic removal rates with aluminum-based coagulants and polyacrylamide dosing on polyester and polyethylene microplastics. It points out the potential of chemical treatment, but many more studies are required to fine-tune these methods to gain even better results. Based on its review, PAM combined with aluminum- or iron-based coagulants is capable of removing as high as 99% of microplastics, depending on size, quantity, and aquatic parameters. The study also established that electrocoagulation can be an effective method for eliminating plastic particles.
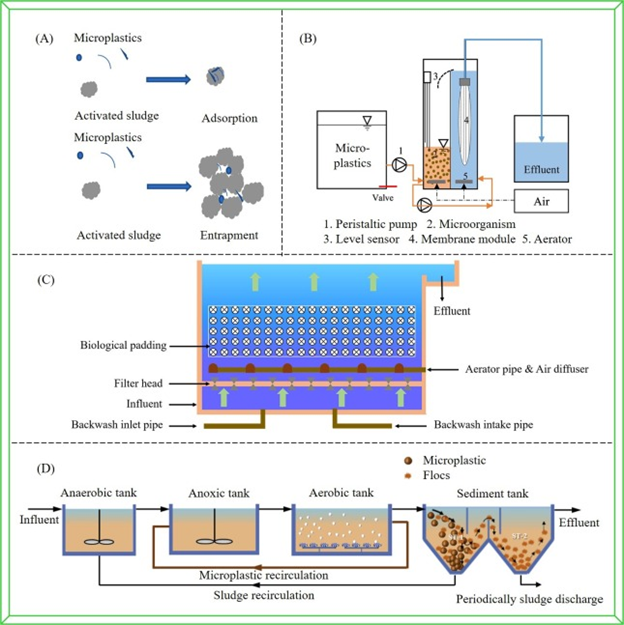
Figure 3: Schematic of Bioreactor system in microplastics removal. (A) Activated sludge process (Zhang et al., 2020); (B) MBR (Adapted from Li et al., 2020a); (C) Biofilter; (D) A20 (Liu et al., 2020b).
Source: Liu et al. (2021)
Source: Liu et al. (2021)
Biotic removal technique
Biological methods for the removal of microplastics, such as processes involving activated residue remedy, aerobic and anaerobic breakdown, or lakes and sewage disposal, appeared studied, but most of them usually have limited efficiency. For instance, the activated sludge systems are based on bacteria that capture microplastics smaller than 0.5 mm, though they normally trap the particles due to a short residence time in effluents and do not degrade them. According to research, virgin microplastics had no significant impact on the activity of key bacterial groups associated with ammonia oxidation and phosphorus accumulation. Other studies, for instance, have documented some degree of microplastic removal or alteration in the growth rate of organisms upon exposure to microplastics (Huang et al., 2020). Most of these biological approaches, however, result in low removal efficiency and can further cause secondary contamination through sludge or sedimentation. As such, the effectiveness of biological methods in removing microplastics needs to be carefully weighed since they are normally very short of providing high removal efficiency.
Biological methods for the removal of microplastics, such as processes involving activated residue remedy, aerobic and anaerobic breakdown, or lakes and sewage disposal, appeared studied, but most of them usually have limited efficiency. For instance, the activated sludge systems are based on bacteria that capture microplastics smaller than 0.5 mm, though they normally trap the particles due to a short residence time in effluents and do not degrade them. According to research, virgin microplastics had no significant impact on the activity of key bacterial groups associated with ammonia oxidation and phosphorus accumulation. Other studies, for instance, have documented some degree of microplastic removal or alteration in the growth rate of organisms upon exposure to microplastics (Huang et al., 2020). Most of these biological approaches, however, result in low removal efficiency and can further cause secondary contamination through sludge or sedimentation. As such, the effectiveness of biological methods in removing microplastics needs to be carefully weighed since they are normally very short of providing high removal efficiency.
Factors Affecting Microplastic Analysis
The substantial amount and dispersal of plastic particles within the water are driven primarily by plastic properties, including hydrophobicity, density, size, and environmental factors such as interactions with organisms, weather conditions, and nearby industrial activities. In the process, plastic waste degrades into finer particles due to exposure to sunlight, waves, and wind. These fine pieces can travel by the action of wind to any part of the world, even finding their way to the remotest of places such as the Alps and Antarctica. Low-density microplastics usually attach to biological materials and deposit, but they are easily resuspended by dynamic weather conditions, leading to redistribution within the water body systems. Mismanagement of industrial wastes is also behind these plastics in these environments. Mismanagement of industrial wastes is also behind these plastics in these environments. Dynamic changes in weather conditions, however, hinder the understanding of the sources and the attribution to microplastics in the water. This will thus make the movement and dispersal of plastic particles both vertically within the water pole and horizontally across different areas greatly influenced by environmental factors, making it hard to measure their presence with just a single sampling. Long-term monitoring is essential to gain a clearer picture. In addition, the inconsistency in mesh sizes used plus the difference in techniques of sampling and processing challenges the consistency in interpreting results. To enhance microplastic analysis, Lusher et al.(2018) suggested that sampling and pretreatment methods should be standardized. Since different reagents applied for separation techniques influence different kinds of plastics, it is necessary to work out specific protocols for each kind of polymer.
The substantial amount and dispersal of plastic particles within the water are driven primarily by plastic properties, including hydrophobicity, density, size, and environmental factors such as interactions with organisms, weather conditions, and nearby industrial activities. In the process, plastic waste degrades into finer particles due to exposure to sunlight, waves, and wind. These fine pieces can travel by the action of wind to any part of the world, even finding their way to the remotest of places such as the Alps and Antarctica. Low-density microplastics usually attach to biological materials and deposit, but they are easily resuspended by dynamic weather conditions, leading to redistribution within the water body systems. Mismanagement of industrial wastes is also behind these plastics in these environments. Mismanagement of industrial wastes is also behind these plastics in these environments. Dynamic changes in weather conditions, however, hinder the understanding of the sources and the attribution to microplastics in the water. This will thus make the movement and dispersal of plastic particles both vertically within the water pole and horizontally across different areas greatly influenced by environmental factors, making it hard to measure their presence with just a single sampling. Long-term monitoring is essential to gain a clearer picture. In addition, the inconsistency in mesh sizes used plus the difference in techniques of sampling and processing challenges the consistency in interpreting results. To enhance microplastic analysis, Lusher et al.(2018) suggested that sampling and pretreatment methods should be standardized. Since different reagents applied for separation techniques influence different kinds of plastics, it is necessary to work out specific protocols for each kind of polymer.
Advancement and Renovation in Microplastic Research
Research on microplastics is rapidly underway as scientists track down new ways to solve this growing environmental problem. Raman spectroscopy uses laser light to identify various plastics by their distinct molecular fingerprinting. Next-generation sequencing reveals just how microplastics are impacting the environment by decoding the DNA of organisms that come in contact with them. Portable biosensors—for example, light-emitting bacteria or enzymes in the presence of specific plastics—can provide coverage for a wide range of on-site rapid detection. Small devices, such as microfluidic platforms, are involved in the processing and analysis of vastnumbers of samples with high efficiency, while high-resolution microscopy offers identification through detailed images of the microplastics and hence their sources and potential dangers.
Research on microplastics is rapidly underway as scientists track down new ways to solve this growing environmental problem. Raman spectroscopy uses laser light to identify various plastics by their distinct molecular fingerprinting. Next-generation sequencing reveals just how microplastics are impacting the environment by decoding the DNA of organisms that come in contact with them. Portable biosensors—for example, light-emitting bacteria or enzymes in the presence of specific plastics—can provide coverage for a wide range of on-site rapid detection. Small devices, such as microfluidic platforms, are involved in the processing and analysis of vastnumbers of samples with high efficiency, while high-resolution microscopy offers identification through detailed images of the microplastics and hence their sources and potential dangers.
Monitoring devices, such as adsorption pads or artificial mussels, have been used to provide a record of microplastic accumulation over time across multiple situations recently. There exists the hope of being able to clean up existing pollution from research into microbes and enzymes that can degrade plastics. To prevent more microplastics from entering our environment in the first place, developing biodegradable alternatives and enhancing waste management (in particular, recycling) are pressing tasks. Global efforts by the International Microplastics Collaborative and others, such as the United Nations, are very helpful in sharing data and coordinating research among regions, according to Jambeck et al. (2015). This brings together a wide range of experts, from engaging the public with education and community science in the search for effective solutions.
Challenges Posed By Microplastics and Effective Solutions
The increased awareness of the microplastics' impact on the environment shows the direct need for additional research into the matter. Although plastics are being continuously produced and disposed of, there is a lack of effective waste management. The plastics, once let out into the environment, break down into small particles that could enter the food chain through small organisms and eventually pose risks to human health. It can be a serious threat that cannot be disregarded, considering that microplastics detected in the lungs and digestive human system have been reported by Jenner et al. (2021). Microplastics smaller than 1.5 μm are very dangerous because they may penetrate cell membranes, leading to a direct damage effect on cells. In-situ analysis, where samples are analyzed in their natural environment, is recommended to avoid contamination.
The increased awareness of the microplastics' impact on the environment shows the direct need for additional research into the matter. Although plastics are being continuously produced and disposed of, there is a lack of effective waste management. The plastics, once let out into the environment, break down into small particles that could enter the food chain through small organisms and eventually pose risks to human health. It can be a serious threat that cannot be disregarded, considering that microplastics detected in the lungs and digestive human system have been reported by Jenner et al. (2021). Microplastics smaller than 1.5 μm are very dangerous because they may penetrate cell membranes, leading to a direct damage effect on cells. In-situ analysis, where samples are analyzed in their natural environment, is recommended to avoid contamination.
Although there is a massive research interest in the toxicity of microplastics, the smallness of their size is still a considerable challenge for their correct evaluation. Microplastics may have hazards not only due to physical and chemical characteristics but also because of carrying some toxic chemicals from the environment. Most of the current studies are oriented to virgin plastic materials, however, aging may change the chemical characteristics of microplastics because of environmental factors. Such changes need further investigation to know their impacts on their toxicity. Plastic wastes continue to fragment and pose threats to life on Earth. There is a clear need for strategies for managing waste so that the uncontrolled release of plastic waste stops. Such accurate research on microplastics requires standardized methods of sample collection and pretreatment and advanced techniques to identify them at their nanoscale level. It also needs research on the toxicity of microplastics with contaminants attached to them and all environmental factors that may affect them. In addition, information on the behavior, entrance to the human body, and minimization of microplastic concentration are vital. If it is not possible to completely avoid plastic waste, then at least research should be done on the safe levels of microplastics in the human body.
Conclusion
Due to their non-biodegradable nature, plastics contaminate a large portion of the environment, and the contamination is increasing as production increases globally; therefore, microplastics are considered by many to be a universal challenge. The review discusses the dispersal, harmful effects, evaluation methods, and elimination approach connected to plastic particles. The major contaminants are regular plastics, while sources range from agricultural and industrial wastewater to cosmetic products and road runoff. Microplastics are ingested through drinking water, rivers, seas, soil, and air, and they are a potential danger to health. Their analytical process includes sampling, pre-treatment, and analytical approach. This review considers removal techniques in WWTPs, both physical and chemical, together with biological methods; the removal values after secondary treatment amount to more than 70% in sewage remedy techniques. Despite this progress, more comprehensive research is urgently needed about national policies for tackling microplastic contamination.
Acknowledgements
The authors acknowledge the Departments of Chemistry and Industrial Chemistry of Ekiti State University, Ado-Ekiti, Nigeria for their support.
The authors acknowledge the Departments of Chemistry and Industrial Chemistry of Ekiti State University, Ado-Ekiti, Nigeria for their support.
ORCID: Olayinka Abidemi Ibigbami http://orcid.org/0000-0002-4726-6960
References
- Abeynayaka, A., F. Kojima, Y. Miwa, N. Ito, Y. Nihei, Y., Fukunaga, Y., Yashima, Y., & N. Itsubo, (2020). Rapid Sampling of Suspended and Floating Microplastics in Challenging Riverine and Coastal Water Environments in Japan. Water Res, 12: 1903.
- Al-Azzawi, M. S. M., S. Kefer, J. Weiber, J., Reichel, C. Schwaller, K. Glas, O. Knoop & . J. E Drewes, (2020). Validation of Sample Preparation Methods for Microplastic Analysis in Wastewater Matrices-Reproducibility and Standardization. Water Res, 12, 24-45.
- Asamoah, B. O., B. Kanyathare, M. Russey, & K.E. Peiponen, (2019). A prototype of a portable optical sensor for the detection of transparent and translucent microplastics in freshwater. Chemosphere, 231, 161–167.
- Barnes, D. K. A., F. Galgani, R.C Thompson, & M. Barlaz, (2009). Accumulation and fragmentation of plastic debris in global environments. Philos. Trans. R. Soc. B Biol. Sci, 364, 1985–1998.
- Barrows, A. P. W., C.A. Neumann, M.L. Berger & S. D. Shaw (2017). Grab vs. neuston tow net: A microplastic sampling performance comparison and possible advances in the field. Anal. Methods, 9, 1446 –1453.
- Besseling, E., P. Redondo-Hasselerharm, E. M. Foekema, & A. A. Koelmans, (2019). Quantifying ecological risks of aquatic micro-and nanoplastic. Crit. Rev. Environ. Sci. Technol, 49, 32–80.
- Bhatnagar, A., & I. Anastopoulos (2017). Adsorptive removal of bisphenol A (BPA) from aqueous solution: A review. Chemosphere, 168, 855–902.
- Bratton, S. P., J. Halbur, K. Schreiner, E. C. Minor, E. Hendrickson, & C. A. Peters. (2018). Pyr-GC/MS analysis of microplastics extracted from the stomach content of benthivore fish from the Texas Gulf Coast. Mar. Pollut. Bull, 137, 91–95.
- Campanale, C., I. Savino, I. Pojar, C. Massarelli, & V.F. Uricchio. (2020). A Practical Overview of Methodologies for Sampling and Analysis of Microplastics in Riverine Environments. Sustainability, 12, 6755.
- Carr, S. A., J. Liu & A.G. Tesoro. (2016). Transport and fate of microplastic particles in wastewater treatment plants. Water Res,91, 174–182.
- Conley, K., A. Clum, J. Deepe, H. Lane & B. Beckingham, (2019). Wastewater treatment plants as a source of microplastics to an urban estuary: Removal efficiencies and loading per capita over one year. Water Res, 3, 100030.
- Corradini, F., P. Meza, R. Equiluz, F. Casado, E. Huerta-Lwanga & V. Geissen. (2019). Evidence of microplastic accumulation in agricultural soils from sewage sludge disposal. Sci. Total Environ, 671, 411–420.
- Cutroneo, L., A. Reboa, G., Besio, F. Borgogno, L. Canesi, S. Canuto, M. Dara, F. Enrile, I. Forioso & G. Greco. (2020). Microplastics in seawater: Sampling strategies, laboratory methodologies, and identification techniques applied to the port environment. Environ.Sci. Pollut. Res, 27, 8938–8952.
- Dioses-Salinas, D. C., Pizarro-Ortega, C. I., & De-la-Torre, G. E. (2020). A methodological approach of the current literature on microplastic contamination in terrestrial environments: Current knowledge and baseline considerations. Sci. Total Environ, 730, 139164.
- Dris, R., J. Gasperi, V. Rocher, M. Saad, N. Renault & B. Tassin, B. (2015). Microplastic contamination in an urban area: A case study in Greater Paris. Environ. Chem, 12, 592–599.
- Dümichen, E., A. K. Barthel, U. Braun, C. G. Bannick, K. Brand, M. Jekel & R. Senz. (2015). Analysis of polyethylene microplastics in environmental samples, using a thermal decomposition method. Water Res, 85, 451-457.
- Edo, C., M. González-Pleiter, F. Leganés, F. Fernández-Piñas & R. Rosal (2020). Fate of microplastics in wastewater treatment plants and their environmental dispersion with effluent and sludge. Environ. Pollut,259, 113-837.
- Efimova, I., M. Bagaeva, A. Bagaev, A., Kileso & I.P Chubarenko, (2018). Secondary Microplastics Generation in the Sea Swash Zone with Coarse Bottom Sediments: Laboratory Experiments. Front. Mar. Sci, 5, 313.
- Funck, M., A. Yildirim, C. Nickel, J. Schram, T. C Schmidt & J. Tuerk, (2020). Identification of microplastics in wastewater after cascade filtration using Pyrolysis-GG-MS. Methods X, 7, 100778.
- Galloway, T., M. Cole & C. Lewis, (2017). Interactions of microplastic debris throughout the marine ecosystem, 1, 0116.
- Gao, F., J. Li, C. Sun, L. Zhang, F. Jiang, W. Cao & L. Zheng, L. (2019). Study on the capability and characteristics of heavy metals enriched on microplastics in marine environments. Mar. Poll. Bull, 144, 61–67.
- Harris, P. T. (2020). Review: The fate of microplastic in marine sedimentary environments: A review and synthesis. Mar. Poll. Bull, 158, 111-398.
- Hebner, T. S., & M. A. Maurer-Jones. (2020). Characterizing microplastic size and morphology of photodegraded polymers placed in simulated moving water conditions. Environ. Sci. Process. Impacts, 22, 398–407.
- Hendrickson, E., E.C. Mino & K. Schreiner. (2018). Microplastic abundance and composition in western Lake Superior as determined via microscopy, Pyr-GC/MS, and FTIR. Environ. Sci. Technol, 52, 1787-1796.
- 25.Huang, Y., Q. Liu, W. Jia, C. Yan, & J. Wang. (2020). Agricultural plastic mulching as a source of microplastics in the terrestrial environment. Environ. Pollut,260, 114096.
- Isobe, A.,S. Iwasaki, K. Uchida & T. Tokai (2019). Abundance of non-conservative microplastics in the upper ocean from 1957 to 2066. Nat. Commun, 10, 417.
- Jambeck, J. R., R. Geyer, C. Wilcox, T. R. Siegler, M. Perryman, A. Andrady. R. Narayan, R. & K. L. Law. (2015). Plastic waste inputs from land into the ocean. Science, 347, 768–771.
- Jenner, L. C., E. Sadofsky & E. Danopoulos. (2021). Detection of Microplastic in human lung tissues using μFTIR Spectroscopy. Atmos. Environ, 259, 118-512.
- Jones, J. I., A. Vdovchenko, D. Cooling, J. F. Murphy, A. Arnold, J.L. Pretty, K.L Spencer, A. A. Markus, A. D. Vethaak,& M. Resmini. (2020). Systematic Analysis of the Relative.
- Kaile, N., M. Lindivat, J. Elio, G. Thuestad, Q. G. Crowley &I. A. Hoell. (2020). Preliminary Results From Detection of Microplastics in Liquid Samples Using Flow Cytometry. Front. Mar. Sci, 7, 856.
- Kang, H., S. Park, B. Lee, J. Ahn & S. Kim. (2020). Modification of a Nile Red Staining Method for Microplastics Analysis: A Nile Red Plate Method. Water, 12, 3251.
- Käppler, A., D. Fischer, S. Oberbeckmann, G. Schernewski, M. Labrenz, K.J. Eichhorn & B. Voit. (2016). Analysis of environmental microplastics by vibrational microspectroscopy: FTIR, Raman or both? Anal. Bioanal. Chem, 408, 8377-8391.
- Kim, H. J. & J. Y. & Lee. (2020). Emerging Concerns about Microplastic Pollution on Groundwater in South Korea. Sustainability, 12, 5275.
- Kunz, A., B. A. Walther, L. Lowemark, & Y. C. Lee. (2016). Distribution and quantity of microplastic on sandy beaches along the northern coast of Taiwan. Mar. Pollut. Bull, 111: 126–135.
- Lapointe, M., J. M. Farner, L. M.Hernandez, & N. Tufenkji. (2020). Understanding and Improving Microplastic Removal during Water Treatment: Impact of Coagulation and Flocculation. Environ. Sci. Technol, 54, 8719–8727.
- Lenaker, P. L., A.K. Baldwin, S. R .Corsi, S. A. Mason, P. C. Reneau & J. W. Scott. (2019). Vertical Distribution of Microplastics in the Water Column and Surficial Sediment from the Milwaukee River Basin to Lake Michigan. Environmental Science and Technology 53 (21): 12227-12237.
- Lindeque, P. K., Cole, M., Coppock, R. L., Lewis, C. N., Miller, R. Z., Watts, A. J. R., Wilson-McNeal, A., Wright, S. L., & Galloway, T. S. (2020). Are we underestimating microplastic abundance in the marine environment? A comparison of microplastic capture with nets of different mesh sizes. Environ. Pollut, 265, 114-721.
- Liu, C., J. Li, Y. Zhang, L. Wang, J. Deng, Y. Gao, L. Yu, J. Zhang & H. Sun. (2019). Widespread distribution of PET and PC microplastics in dust in urban China and their estimated human exposure. Environ. Int, 128, 116–124.
- Liu, F., N. B. Nord, K. Bester, & J. Vollertsen, (2020a). Microplastics removal from treated wastewater by a biofilter, 12 p. 1085.
- Liu, W.L., Y. Wu, J. S. Zhang, Y. O Gao, Y. Jiang, H. Horn & J. Li. (2020b). Successufl granulation and microbial differentiation of activated sludge in anaerobic /anoxic/aerobic (A20) reactor with two-zone sedimentation tank treating municipal sewage. Water Res. 178: 115825
- Liu, W., J. Zhang. H. Liu, X. Guo, X. Zhang, X. Yao, Z. Cao, & T. Zhang. (2021). A review of the removal of microplastics in global wastewater treatment plants: Characteristics and mechanism. Environ. Inter. 146, 106277
- Lusher, A. L., K. Munno, L. Hermabessiere, & S. Carr. (2020). Isolation and Extraction of Microplastics from Environmental Samples: An Evaluation of Practical Approaches and Recommendations for Further Harmonization. Appl. Spectrosc, 74, 1049–1065.
- Mason, S. A., V.G. Welch & J. Neratko (2018). Synthetic Polymer Contamination in Bottled Water. Front. Chem, 6, 407.
- McCormick, A. R, T.J. Hoellein, M. G. London, J. Hittie, J.W. Scott, & J. J. Kelly. (2016). Microplastic in Surface Waters of Urban Rivers: Concentration, Sources, and Associated Bacterial Assemblages. Ecosphere 7, e01556
- Müller, A., C. Goedecke, P. Eisentraut, C. Piechotta & U. Braun, U. (2020). Microplastic analysis using chemical extraction followed by LC-UV analysis: A straightforward approach to determine PET content in environmental samples. Environ. Sci. Eur, 32, 85.
- Ockelford, A., A. Cundy & J.E. Ebdon. (2020). Storm Response of Fluvial Sedimentary Microplastics. Sci. Rep, 10, 1865.
- Pan, Z., H. Guo, H. Chen, S. Wang, X. Sun, Q. Zou, Y. Zhang, H. Lin, S. Cai, & J. Huang. (2019). Microplastics in Northwestern Pacific: Abundance, distribution, and characteristics. Sci. Total Environ, 650, 1913–1922.
- Plastics Europe. Plastics the Facts (2019): An Analysis of European Plastics Production, Demand and Waste Data. Plastics Europe, Brussels. 2019. Available online: https://www.plasticseurope.org/application/files/9715/7129/9584/FINAL_web_version_Pollut. Res, 27, 8938–8952.
- Pop, C. E., S. Draga, R. M?ciuc?, R. Nit, N. Cr?ciun & R. Wolff R. (2021). Bisphenol A Effects in Aqueous Environment on Lemna minor. Processes, 9, 1512.
- Primpke, S., S. H. Christiansen, K.J. Wiggin. (2020). Critical assessment of analytical methods for the harmonized and cost efficient analysis of microplastics. Applied Spectroscopy 74 (9): 1012 - 1047.
- Primpke, S., R.K. Cross, S. M. Mintenig, M. Simon, A. Vianello, G. Gerdts& J. Vollertsen. (2020). Toward the systematic identification of MPs in the environment: Evaluation of a new independent software tool (simple) for spectroscopic analysis. Appl. Spectrosc, 74.
- Renner, G., T.C. Schmidt & J. Schram. (2018). Analytical methodologies for monitoring micro (nano) plastics: Which are fit for purpose? Curr. Opin. Environ. Sci.Health, 1, 55-61.
- Rocha-Santos, T, & A.C. Duarte. (2015). A critical overview of the analytical approaches to the occurrence, the fate, and the behavior of microplastics in the environment. Trends Anal. Chem, 65, 47–53.
- Russell, M., & L. Webster. (2016). Microplastics in sea surface waters around Scotland. Mar. Pollut. Bull,116, 112210.
- Scircle, A., J. V. Cizdziel, L. Tisinger, T. Anumol, & D. Robey. (2020). Occurrence of Microplastic Pollution at Oyster Reefs and Other Coastal Sites in the Mississippi Sound, USA: Impacts of Freshwater Inflows from Flooding. Toxics. 8, 35.
- Stock, V., L. Böhmert, E. Lisicki, R. Block, J. Cara-Carmona, L.K Pack, R. Selb, D. Lichtenstein, L. Voss & C.J. Henderson. (2019). Uptake and effects of orally ingested polystyrene microplastic particles in vitro and in vivo. Arch. Toxicol, 93, 1817–1833.
- SubCtech GmbH (2020). Subsea Technologies for the Marine Environment (Microplastic Sampler). Available online: https://subctech.com/wp-content/uploads/2020/09/SpecSheet_SubCtech_Microplastic-Sampler_ENG_v1-0.pdf (accessed on 23 June 2021).
- Sun, J., X. Dai, Q. Wang, M.C.M. van Loosdrecht, & B.J. Ni. (2019). Microplastics in wastewater treatment plants: Detection, occurrence and removal. Water Res, 152, 21–37.
- Syakti, A.D., N.V. Hidayati, Y.V, Jaya, S.H. Siregar, R. Yude et al. (2018) Simultaneous Grading of Microplastic Size Sampling in the Small Islands of Bintan Water, Indonesia. Marine Pollution Bulletin, 137, 593-600.
- Tagg,A.S., M. Sapp,J. P, Harrison & J.J. Ojeda. (2015) Identification and Quantification of Microplastics in Wastewater Using Focal Plane Array-Based Reflectance Micro-FT-IR Imaging. Anal. Chem. 2015, 87, 6032–6040.
- Thomas, D., B. Schütze, M. W, Heinze & Z. Steinmetz. (2020). Sample Preparation Techniques for the Analysis of Microplastics in Soil. A Review. Sustainability, 12, 9074.
- Tibbetts, J., S. Krause, I. Lynch, & G.H.S. Smith. (2018). Abundance Distribution and Divers of Microplastic Contamination in Urban River Environments. Water,10, 1597.
- Vogelsang, C., A. Lusher, I. Sundvor & M. Umar (2018). Microplastics in Road Dust-Characteristics, Pathways and Measures; Technical Report, M-959. Norwegian Institute for Water Research: Oslo, Norway.; Available online: https://www.miljodirektoratetno/globalassets/publikasjoner/M959/M959.pdf (accessed on 10 July 2021).
- Von Ammon, U., A. Jeffs, A Zaiko, A. van der Resi, D. Goodwin, L.E. Beckley, E. Malpot, & X. Pochon. (2020). A Portable Cruising Speed Net: Expanding Global Collection of Sea Surface Plankton Data. Front. Mar. Sci, 7, 1109.
- Wang, W., A.W. Ndungu, Z. Li & J. Wang, J. (2017). Microplastics pollution in inland freshwaters of China: A case study in urban surface waters of Wuhan, China. Sci. Total Environ, 575, 1369–1374.
- Wang, T., L. Wang, Q. Chen, N. Kalogerakis, R. Ji, & Y. Ma, (2020). Interactions between microplastics and organic pollutants: Effects on toxicity, bioaccumulation, degradation, and transport. Sci. Total Environ,748, 142-427.
- Webber, A., M. A.L Van Randow, A.L. Voigt, M. Van der Au, E. Fischer, B. Meermann & M. Wagner. (2021). Ingestion and toxicity of microplastics in the freshwater gastropod Lymnaea stagnalis: No microplastic-induced effects alone or in combination with copper. Chemosphere, 263, 128040.
- Willis, K. A., R. Eriksen, C. Wilcox & B.D. Hardesty. (2017). Microplastic Distribution at Different Sediment Depths in an Urban Estuary. Front. Mar. Sci, 4, 419.
- Xu, J. L., K.V. Thomas, Z. Luo & A. A. Gowen. (2019). FTIR and Raman imaging for microplastics analysis: State of the art, challenges and prospects. Trac Trend.Anal.Chem, 119, 115629.
- Xu, K., Y. Zhang, Y. Huang & J. Wang. (2021). Toxicological effects of microplastics and phenanthrene on zebrafish (Danio rerio). Sci. Total Environ, 757, 143730.
- Yukioka, S., S. Tanaka, Y. Nabetani, Y. Suzuki, T. Ushijima, S. Fujii, H. Takada, Q. Van Tran, Q. & S. Singh. (2020). Occurrence and characteristics of microplastics in surface road dust in Kusatsu (Japan), Da Nang (Vietnam), and Kathmandu (Nepal). Environ Pollut, 256: 113447.
- Zhang, K., A.H Hamidian, A. Tubic ?, Y. Zhang, J. K. H. Fang, C. Wu. & P.K.S. Lam. (2021). Understanding plastic degradation and microplastic formation in the environment: A review. Environ. Pollut,274, 116554.
- Zhou R., G. Lu, Z. Yan, R. Jiang, X. Bao & P. Lu. (2020). A review of the influences of microplastics on toxicity and transgenerational effects of pharmaceutical and personal care products in the aquatic environment. Sci. Total Environ, 732, 139222.
Citation: Olayinka Abidemi Ibigbami, Menewoua Mervine Achu and Samuel Sunday Asaolu. (2025). Microplastic Distribution, Removal Techniques and Analysis: A Review. Journal of Pharmacy and Drug Development 7(1).
Copyright: © 2025 Olayinka Abidemi Ibigbami. This is an open-access article distributed under the terms of the Creative Commons Attribution License, which permits unrestricted use, distribution, and reproduction in any medium, provided the original author and source are credited.