Research Article
Volume 3 Issue 1 - 2021
Curcumin and Afatinib Synergistically Inhibit Growth of Human Osteosarcoma Cells by Inhibition of Matrix Metallo Proteinases, Mitogen Activated kinases 1-4, and Reactive Oxygen Species
1Professor of Pharmaceutical Sciences, Union University College of Pharmacy, 1050 Union University Drive Jackson, TN 38305, United States
2Postdoctoral Researcher, Rutgers Biomedical and Health Sciences, RWJ University Hospital New Brunswick, NJ, 08901, United States
2Postdoctoral Researcher, Rutgers Biomedical and Health Sciences, RWJ University Hospital New Brunswick, NJ, 08901, United States
*Corresponding Author: Lunawati L Bennett, Professor of Pharmaceutical Sciences, Union University College of Pharmacy, 1050 Union University Drive Jackson, TN 38305, United States.
Received: June 30, 2021; Published: July 21, 2021
Abstract
Osteosarcoma (OS) is the most common form of bone cancer in humans, primary affecting children and adolescence. OS can metastasis to lung and other bones. The five years’ survival rate of OS are only about 55-70% and if metastases is less than 20%. Chemotherapeutic drugs have been used but these drugs also caused high toxicity to normal cells; therefore, alternative chemotherapy are needed to possibly use lower amount of these drugs. Natural products with anti-cancer properties may provide synergistic effect along with chemotherapeutic drugs. Present study was aimed to evaluate our hypothesis that the combination of curcumin (CUR), a natural antioxidant, and a lower dose of afatinib (AF), an anti-cancer second generation tyrosine kinase inhibitor (TKI), would have a synergistic effect on human Saos-2 cells based on results from MTT assay, Hoechst 33342, Nuclear ID Red/Green and Rhodamine 123 staining, and Western Blot. Based on different signaling pathway being affected, CUR at 30 ?M enhanced the chemotherapeutic activity of low dose AF at 3 µM in causing cell death as compared to AF alone at high dose. Results from this study showed possible use of low dose AF with CUR to be a treatment option for osteosarcoma.
Keywords: Afatinib; Curcumin; Saos-2; Apoptosis; Osteosarcoma
Abbreviations: CUR: Curcumin; AF: Afatinib; OS: Osteosarcoma; TKI: Tyrosine kinase inhibitor; Apaf-1: Activator protein 1; MAPK: mitogen-activated protein kinase; EGFR: Epidermal growth factor; H2DCFDA: 2', 7’-Dichlorodihydrofluorescein diacetate; Cl. PARP: Cleaved poly (ADP-Ribose) polymerase; ROS: Reactive oxygen species; Casp: Caspase; MMP: Matrix Metallo Proteinase
Introduction
Chemotherapeutic drugs such as doxorubicin, cisplatin, methotrexate, or a combination of bleomycin, cytoxan and actinomycin D have been successfully used to treat cancer such as osteosarcoma (OS), but they can also induce severe side effects and toxicities to normal cells [1]. To overcome this significant problem, novel chemotherapeutic approaches are needed to better treating OS. Decreasing the concentration of chemotherapeutic drugs as low as possible while maintaining their effectiveness to cause cell death to OS, by adding natural products such as curcumin, resveratrol, or others which cause no toxicities to normal cells, can provide an ideal solution.
Curcumin (CUR), an active compound derived from the rhizome plant Curcuma longa, has been identified of having anti-cancer properties in colorectal, pancreatic, lung, breast and hematological malignancies [2-7]. Previous reports demonstrated that the chemo-preventive activity of CUR involves the regulation of various oncogenic molecules and pathways such as activator protein 1 (apaf-1), mitogen-activated protein kinase (MAPK), cyclin D1, EGFR, and others [8-9]. Moreover, CUR has been shown in various preclinical trials to prevent or to treat multiple myeloma, colorectal or pancreatic cancers, and familial adenomatous polyposis, alone or in combination with anti-cancers such as capecitabine, gemcitabine, or use of radiation radiotherapy [10].
Osteosarcoma (OS) is the third most common cancer of bone and joint affecting children and adolescents. This cancer has an annual incidence of 5.6 cases per million children under the age of 15, with peak incidence around the second decade of life [11-14]. According the National Cancer Institute, there is an estimated of 3600 new cases of OS with estimated death of 1720 and five years’ survival rate of 66% in 2020 in the United States [15]. Worldwide incidence of OS is about 3.4 people per million per year [16]. Treatment of OS include surgical amputation, radiation, and chemotherapy. Radiation treatment has a controversial issue due to its questionable effectiveness and possible increased risk of infection [14]. Additionally, there is only about 55% disease –free cases after patients go through a two years of treatment with chemotherapeutics drugs [1].
Afatinib (AF) is the second generation TKI that function to selectively inhibit epidermal growth factor (EGFR) and human epidermal growth factor receptor 2 (HER-2/neu) [17-19]. This TKI was approved by the Food and Drug Administration for the treatment of patients with EGFR-mutated non-small cell lung cancer. This drug inhibits downstream oncogenic signaling pathways correlated to cancer proliferation, invasion, metastasis and angiogenesis [17-20]. It is irreversibly bind to ErbB family blocking signaling of EGFR family dimers [21-22]. Since treatment of cancer with AF monotherapy can cause drug resistance to EGFR and other oncogenic proteins; there is a need to find effective adjuvant therapy that can limit AF resistance and toxicities with long lasting remission or cure effect [23].
This investigation was designed to evaluate the chemo-enhancing potential of CUR in combination with low dose AF for the treatment of human OS using Saos-2 cells line as a model. To our knowledge, this study is the first to report inhibition of cancer-signaling related pathway showing the efficacy of CUR working synergistically with low dose of AF in the treatment of human OS.
Materials and Methods or Experimental Procedures
Chemicals
Curcumin, 3- [4, 5-dimethylthiazol-2-yl] -2, 5-diphenyl-tetrazolium bromide (MTT), RIPA buffer, protease inhibitor, and phosphatase inhibitor were purchased from Sigma (Saint Louis, MO, USA). Afatinib was obtained from LC Laboratories (Woburn, MA, USA). NucBlueTM live cell stain Hoechst 33342 was purchased from Life Technologies (Carlsbad, CA, USA), H2DCFDA (2', 7’-Dichlorodihydrofluorescein diacetate) from Invitrogen (Eugene, OR, USA), Nuclear-ID red/green cell viability reagent from Enzo Life Sciences Inc (Farmingdale, NY, USA), respectively. Bradford reagent and Enhanced Chemi luminescense (ECL) detection kit were supplied by Bio-Rad (Hercules, CA, USA). Dimethyl sulfoxide (DMSO) was purchased from Amresco (Solon, OH, USA). Cl. poly (ADP-Ribose) polymerase (PARP), casp-3, cl. casp-3, casp-9, cyclin D1, cyclin B1, cytochrome C, MMP2, MMP9, and CDK2 were purchased from Cell Signaling Technology (Beverley, MA, USA). Dry milk, b-actin, Bax, Bcl2, Apaf-1, CDK7, MEK-1 to -4, were purchased from Santa Cruz Biotechnology, Inc (Santa Cruz, CA, USA).
Curcumin, 3- [4, 5-dimethylthiazol-2-yl] -2, 5-diphenyl-tetrazolium bromide (MTT), RIPA buffer, protease inhibitor, and phosphatase inhibitor were purchased from Sigma (Saint Louis, MO, USA). Afatinib was obtained from LC Laboratories (Woburn, MA, USA). NucBlueTM live cell stain Hoechst 33342 was purchased from Life Technologies (Carlsbad, CA, USA), H2DCFDA (2', 7’-Dichlorodihydrofluorescein diacetate) from Invitrogen (Eugene, OR, USA), Nuclear-ID red/green cell viability reagent from Enzo Life Sciences Inc (Farmingdale, NY, USA), respectively. Bradford reagent and Enhanced Chemi luminescense (ECL) detection kit were supplied by Bio-Rad (Hercules, CA, USA). Dimethyl sulfoxide (DMSO) was purchased from Amresco (Solon, OH, USA). Cl. poly (ADP-Ribose) polymerase (PARP), casp-3, cl. casp-3, casp-9, cyclin D1, cyclin B1, cytochrome C, MMP2, MMP9, and CDK2 were purchased from Cell Signaling Technology (Beverley, MA, USA). Dry milk, b-actin, Bax, Bcl2, Apaf-1, CDK7, MEK-1 to -4, were purchased from Santa Cruz Biotechnology, Inc (Santa Cruz, CA, USA).
Growth media andCell culture
The human osteosarcoma Saos-2 cell was cultured in McCoy’s 5A medium, supplemented with 10% fetal bovine serum (FBS), 1% streptomycin/penicillin, which all were purchased from ATCC (Manassas, VA, USA). The cells were maintained in incubator at 37ºC in 5% CO2 humidified environment. Cell culture dishes (T75) were purchased from Greiner-Bio One (Monroe, NC, USA).
The human osteosarcoma Saos-2 cell was cultured in McCoy’s 5A medium, supplemented with 10% fetal bovine serum (FBS), 1% streptomycin/penicillin, which all were purchased from ATCC (Manassas, VA, USA). The cells were maintained in incubator at 37ºC in 5% CO2 humidified environment. Cell culture dishes (T75) were purchased from Greiner-Bio One (Monroe, NC, USA).
Cell viability assay
Cell viability was assessed by MTT method previously described [24]. Briefly, Saos-2 cells were plated in a 96-well microplate dish at 1x105 cells/ml (Greiner-Bio One, Monroe, NC, USA) and incubated overnight to allow the cells to adhere to the wells, followed by treatment of cells with a series concentrations of AF, CUR, or their combination. After 24 h of incubation at 37ºC in 5% CO2, 50 µl MTT solution (2mg/ml) was added to each wells followed by four hours of incubation at 37ºC in 5% CO2 after which 150 ml DMSO was added to dissolve violet blue crystals. The cell viabilities were determined by measuring the absorbance at 570 nm with spectrophotometer (Molecular Devices, CA, USA).
Cell viability was assessed by MTT method previously described [24]. Briefly, Saos-2 cells were plated in a 96-well microplate dish at 1x105 cells/ml (Greiner-Bio One, Monroe, NC, USA) and incubated overnight to allow the cells to adhere to the wells, followed by treatment of cells with a series concentrations of AF, CUR, or their combination. After 24 h of incubation at 37ºC in 5% CO2, 50 µl MTT solution (2mg/ml) was added to each wells followed by four hours of incubation at 37ºC in 5% CO2 after which 150 ml DMSO was added to dissolve violet blue crystals. The cell viabilities were determined by measuring the absorbance at 570 nm with spectrophotometer (Molecular Devices, CA, USA).
Morphological analysis
The appearance of Saos-2 cells treated with AF, CUR or their combination at 0 and 24 hr treatment was observed and photographed under an inverted microscope (Motic AE31, Hong Kong). The changes in morphology of Saos-2 cell of the control and the treatment groups were compared.
The appearance of Saos-2 cells treated with AF, CUR or their combination at 0 and 24 hr treatment was observed and photographed under an inverted microscope (Motic AE31, Hong Kong). The changes in morphology of Saos-2 cell of the control and the treatment groups were compared.
Apoptosis assay
To observe the nuclear chromatin morphological changes of Saos-2 cells, NucBlueTM live cell Hoechst 33342 staining assay was performed as previously described [24]. The amount of apoptotic and non-apoptotic cells treated with CUR, AF, or their combination were captured using FLoid cell imaging station (Life Technologies, Carlsbad, CA, USA) and Fluorescence intensities were measured by Image J software (NIH, USA). A histogram was prepared to compare the percentage changes of apoptotic cells from different treatment groups.
To observe the nuclear chromatin morphological changes of Saos-2 cells, NucBlueTM live cell Hoechst 33342 staining assay was performed as previously described [24]. The amount of apoptotic and non-apoptotic cells treated with CUR, AF, or their combination were captured using FLoid cell imaging station (Life Technologies, Carlsbad, CA, USA) and Fluorescence intensities were measured by Image J software (NIH, USA). A histogram was prepared to compare the percentage changes of apoptotic cells from different treatment groups.
Intracellular ROS assay
To compare intracellular ROS generation in Saos-2 cells after individual AF, CUR, or their combination treatment, H2DCF-DA staining assay was performed following the manufacturer protocol as previously reported [24]. The relative intensities of green fluorescence from different treated groups were captured using FLoid cell imaging station and Fluorescence intensities were measured by Image J software. A histogram was prepared to compare the percentage changes of generated ROS from different treatment groups.
To compare intracellular ROS generation in Saos-2 cells after individual AF, CUR, or their combination treatment, H2DCF-DA staining assay was performed following the manufacturer protocol as previously reported [24]. The relative intensities of green fluorescence from different treated groups were captured using FLoid cell imaging station and Fluorescence intensities were measured by Image J software. A histogram was prepared to compare the percentage changes of generated ROS from different treatment groups.
Mitochondrial membrane potential (?m) assay
To identify the mitochondrial membrane potential changed from different treatment groups, cells were stained with Rhodamine 123 fluorescence probe as previously described [24]. The relative intensities of green fluorescence were captured using FLoid cell imaging and a histogram was prepared to compare relative fluorescence intensities measured by Image J software.
To identify the mitochondrial membrane potential changed from different treatment groups, cells were stained with Rhodamine 123 fluorescence probe as previously described [24]. The relative intensities of green fluorescence were captured using FLoid cell imaging and a histogram was prepared to compare relative fluorescence intensities measured by Image J software.
Wound-healing assay
To asses if AF, CUR or their combination could stop cellular migration, cells at 1x 105 were grown in a 12 well dish. After 24 h, the monolayer Saos-2 cells at confluent of about 70-80% were scratch using a sterile pipette tip, followed by cells treatment with individual AF, CUR or combination of AF and CUR for 24 h incubation in 37°C. The area of migration was photographed (40X) under inverted microscope and the width of the scratch was analyzed at 0 h and 24 h.
To asses if AF, CUR or their combination could stop cellular migration, cells at 1x 105 were grown in a 12 well dish. After 24 h, the monolayer Saos-2 cells at confluent of about 70-80% were scratch using a sterile pipette tip, followed by cells treatment with individual AF, CUR or combination of AF and CUR for 24 h incubation in 37°C. The area of migration was photographed (40X) under inverted microscope and the width of the scratch was analyzed at 0 h and 24 h.
Live and Death cells assay
To access if individual AF, CUR or their combination could cause death of Saos-2 deoxynucleic acid (DNA), the nuclear-ID red/green cell viability assay was performed following manufacture protocol. Cells at 1x 105/ml were placed in 12 well dish for 24 h followed by the treatment with individual AF, CUR or their combination for 24 h prior to detection with the fluorescence probe. The simultaneous combination of red and green dye permits determination of live and death cells which was captures using Floid cell imaging and a histogram was prepared to measure the amount of live and death cells.
To access if individual AF, CUR or their combination could cause death of Saos-2 deoxynucleic acid (DNA), the nuclear-ID red/green cell viability assay was performed following manufacture protocol. Cells at 1x 105/ml were placed in 12 well dish for 24 h followed by the treatment with individual AF, CUR or their combination for 24 h prior to detection with the fluorescence probe. The simultaneous combination of red and green dye permits determination of live and death cells which was captures using Floid cell imaging and a histogram was prepared to measure the amount of live and death cells.
Western blot analysis
The expressions of different signaling proteins were analyzed using Western blot technique as previously described [24]. Briefly, RIPA buffer supplemented with protease and phosphatase inhibitors was used to extract proteins from Saos-2 cells treated with individual AF, CUR, or their combination. The protein concentrations were determined using the Bradford Protein Assay Reagent following the manufacture protocol. Equivalent amounts of protein 50 mg were loaded onto 10% polyacrylamide gels, which was separated by electrophoresis, followed by transferred of the gel unto Immuno- Blot PVDF membranes using Trans Blot Turbo (Bio-Rad Laboratories, Hercules, CA, USA) for 30 minutes. The membranes were then blocked for 2 hours in 5% dry milk dissolved in Tris-buffered saline containing 0.1% Tween-20 (TBST) at room temperature. Finally, the membranes were incubated overnight with specific primary antibodies. Membranes were then after washed several times in TBST, followed by incubation for two h with secondary antibodies. The protein bands were developed using ECL Western Blotting detection reagents and the pictures were taken using Bio-Rad ChemiDoc XRS+.
The expressions of different signaling proteins were analyzed using Western blot technique as previously described [24]. Briefly, RIPA buffer supplemented with protease and phosphatase inhibitors was used to extract proteins from Saos-2 cells treated with individual AF, CUR, or their combination. The protein concentrations were determined using the Bradford Protein Assay Reagent following the manufacture protocol. Equivalent amounts of protein 50 mg were loaded onto 10% polyacrylamide gels, which was separated by electrophoresis, followed by transferred of the gel unto Immuno- Blot PVDF membranes using Trans Blot Turbo (Bio-Rad Laboratories, Hercules, CA, USA) for 30 minutes. The membranes were then blocked for 2 hours in 5% dry milk dissolved in Tris-buffered saline containing 0.1% Tween-20 (TBST) at room temperature. Finally, the membranes were incubated overnight with specific primary antibodies. Membranes were then after washed several times in TBST, followed by incubation for two h with secondary antibodies. The protein bands were developed using ECL Western Blotting detection reagents and the pictures were taken using Bio-Rad ChemiDoc XRS+.
Statistic
All the statistical results were expressed as the mean ± SD of three independent sets of experiments. Differences between individual and combination treatment groups were analyzed using Newman-Keuls one-way ANOVA. * P < 0.05, ** P < 0.01 and *** P < 0.001 were considered statistically significant.
All the statistical results were expressed as the mean ± SD of three independent sets of experiments. Differences between individual and combination treatment groups were analyzed using Newman-Keuls one-way ANOVA. * P < 0.05, ** P < 0.01 and *** P < 0.001 were considered statistically significant.
Results
Cell viability and selection of doses
AF or CUR decreased Saos-2 cell viability in a dose dependent manner based on MTT assay. Initially, cells were treated with different concentrations of AF ranging from 0-100 mM, or CUR from 0 – 500 mM. From initial data, CUR 30 mM was selected to be used with different AF concentration to find the lowest possible concentration of AF. Figure 1(a) depicted individual treatment of cells with 3 µM of AF or 30 µM of CUR showed cell viabilities of approximately 65% and 85%, respectively. Combination CUR at 30 µM with serial dilution of AF (0-100 µM) resulted in dose-dependent decreased of cell viabilities. Cells death in the combinational treatment of 3 µM AF and 30 µM CUR was higher than individual AF or CUR treatment. Based on the MTT result, we chose 3 µM of AF, 30 µM of CUR, and their combination for further experimentations.
AF or CUR decreased Saos-2 cell viability in a dose dependent manner based on MTT assay. Initially, cells were treated with different concentrations of AF ranging from 0-100 mM, or CUR from 0 – 500 mM. From initial data, CUR 30 mM was selected to be used with different AF concentration to find the lowest possible concentration of AF. Figure 1(a) depicted individual treatment of cells with 3 µM of AF or 30 µM of CUR showed cell viabilities of approximately 65% and 85%, respectively. Combination CUR at 30 µM with serial dilution of AF (0-100 µM) resulted in dose-dependent decreased of cell viabilities. Cells death in the combinational treatment of 3 µM AF and 30 µM CUR was higher than individual AF or CUR treatment. Based on the MTT result, we chose 3 µM of AF, 30 µM of CUR, and their combination for further experimentations.
CUR and AF combination treatment caused change in cell morphology
The morphology of Saos-2 cells was changed in all treatment groups as compared to the control. Figure 1 (b) showed that control cells were healthy and attached to the culture dish while most cells were round shaped with higher number of cells dead floating in the media in the combination of CUR and AF dish.
The morphology of Saos-2 cells was changed in all treatment groups as compared to the control. Figure 1 (b) showed that control cells were healthy and attached to the culture dish while most cells were round shaped with higher number of cells dead floating in the media in the combination of CUR and AF dish.
CUR and AF combination treatment induced apoptosis
Combination of 3 µM of AF and 30 µM of CUR induced Saos-2 cells to apoptosis more than cells treated with 3 µM of AF or 30 µM of CUR only, as confirmed by Hoechst 33342 staining using fluorescence microscopic images detection system as shown in Figure 1 (c). Nucleus of control cells showed lower fluorescence intensity signifying that these cells were normal and healthy. The cells undergoing apoptosis showed bright blue color due to condensed and fragmented nucleus. In individual AF or CUR treatment, the percentage of brightly stained cells were higher than control. The percentage of brightly stained apoptotic cells was the highest in the combination treatment of CUR and AF. Figure 1 (d) showed the histogram comparing the apoptotic of control, AF, CUR or combination of AF and CUR.
Combination of 3 µM of AF and 30 µM of CUR induced Saos-2 cells to apoptosis more than cells treated with 3 µM of AF or 30 µM of CUR only, as confirmed by Hoechst 33342 staining using fluorescence microscopic images detection system as shown in Figure 1 (c). Nucleus of control cells showed lower fluorescence intensity signifying that these cells were normal and healthy. The cells undergoing apoptosis showed bright blue color due to condensed and fragmented nucleus. In individual AF or CUR treatment, the percentage of brightly stained cells were higher than control. The percentage of brightly stained apoptotic cells was the highest in the combination treatment of CUR and AF. Figure 1 (d) showed the histogram comparing the apoptotic of control, AF, CUR or combination of AF and CUR.
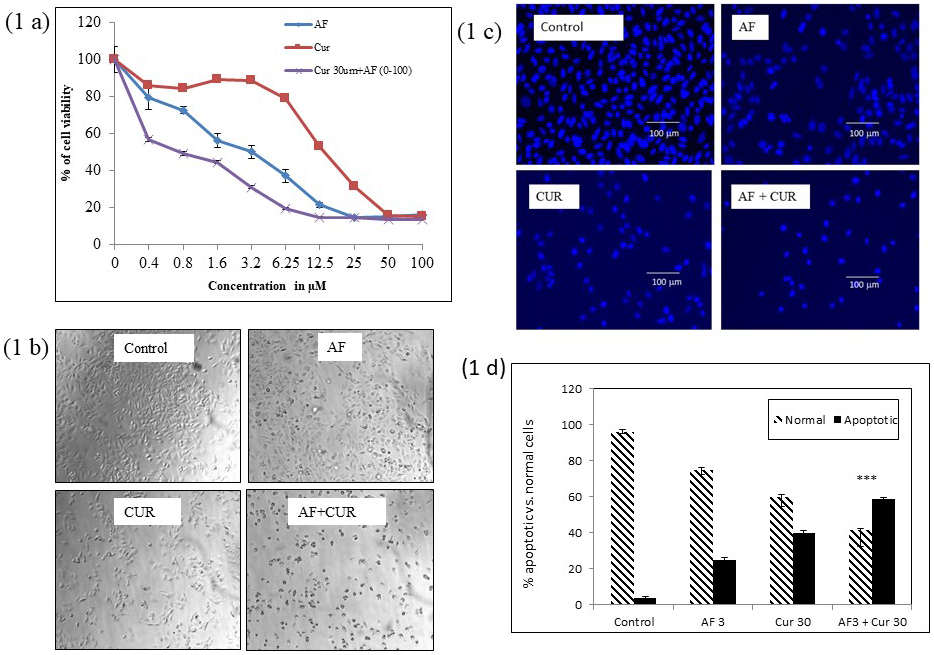
Figure 1: a) MTT assay for cell viability of Saos-2 cells treated for 24 h with different concentrations of AF from 0 – 100 μM, CUR from 0-500 μM, and combination of CUR at 30 μM and serial concentration of AF. 1 (b) Inverted microscopic images of Saos-2 cells after 24 h treatment with AF, CUR, or their combination. Control Saos-2 had normal cell morphology, while cells treated with individual AF or CUR were round shaped. More round shaped cells were detected in cells treated with combination of AF and CUR and these cells were also detached from the flask. Scale bar indicates 100μm. 1 (c) Fluorescence microscopic images of cells after 24 h stained with Hoechst 33342. The number of apoptotic cells (bright nuclei) was slightly increased in individual AF or CUR treatment in comparison to control cells. Highest number of apoptotic cells was observed in Saos-2 cells treated with combination of AF and CUR. Scale bar indicates 100μm. 1 (d) Histogram represented the percentage of apoptotic cells from different treatment group with significantly higher number of apoptotic cells in combination of AF and CUR group (*** = 0.001< p).
CUR and AF combination treatment decreased cell migration
After 24 h of treatment, combination of AF and CUR caused less migration of cells i.e. wider gap of wound than individual AF or CUR treatment in Saos-2 cells. Control showed cells were migrated back closer after 24 h as shown in Figure 2 (a). Cancer cells that migrated back faster and closer after being wounded such as seen in control showed sign of invasion of cancer cells into surrounding tissue and is indication of an initial step in cancer metastasis. Figure 2 (b) showed the histogram comparing the width of wound from Saos-2 control, individual AF or CUR, or combinational treatment.
After 24 h of treatment, combination of AF and CUR caused less migration of cells i.e. wider gap of wound than individual AF or CUR treatment in Saos-2 cells. Control showed cells were migrated back closer after 24 h as shown in Figure 2 (a). Cancer cells that migrated back faster and closer after being wounded such as seen in control showed sign of invasion of cancer cells into surrounding tissue and is indication of an initial step in cancer metastasis. Figure 2 (b) showed the histogram comparing the width of wound from Saos-2 control, individual AF or CUR, or combinational treatment.
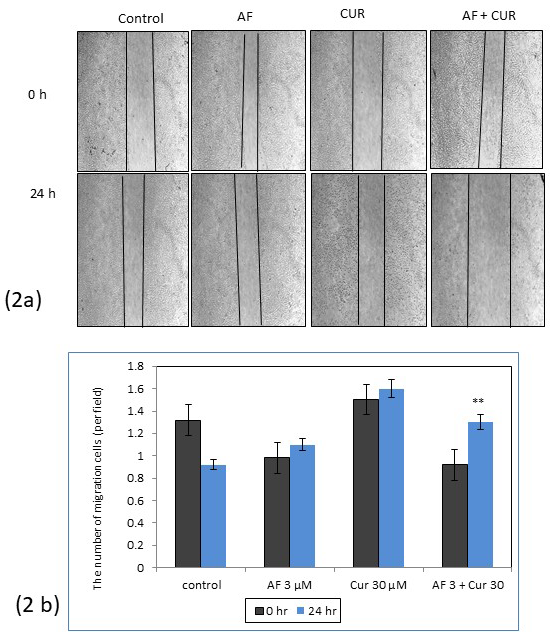
Figure 2: (a) Wound healing assay of Saos-2 cells treated with AF, CUR or combination of AF and CUR in comparison to control at 0 and 24 h. 2 (b) Histogram comparing the width of the wounds from different groups. After 24 h, migration was higher in cells treated with combination of AF and CUR and rounded cells signified apoptosis was noticed in this treatment group (**=0.001 <p).
CUR and AF combination treatment depleted mitochondrial membrane potential
Generation of ROS in the cells indicated cell death due to the depletion of mitochondrial membrane potential. Individual AF, CUR or combination treatment showed induction of cell death as demonstrated with fluorescence microscopic images in Figure 3(a). Control Saos-2 cells had higher fluorescence intensity than the treated cells as shown in histogram Figure 3 (b). Depletion of the fluorescence intensity was significantly higher in combination group than individual AF or CUR treatment. Intracellular ROS formation could also be evaluated with H2DCFDA staining method as seen in Figure 3 (c). Figure 3 (d) histogram intensity showed the intracellular ROS level was significantly higher in the combination of CUR and AF than in individual AF or CUR.
Generation of ROS in the cells indicated cell death due to the depletion of mitochondrial membrane potential. Individual AF, CUR or combination treatment showed induction of cell death as demonstrated with fluorescence microscopic images in Figure 3(a). Control Saos-2 cells had higher fluorescence intensity than the treated cells as shown in histogram Figure 3 (b). Depletion of the fluorescence intensity was significantly higher in combination group than individual AF or CUR treatment. Intracellular ROS formation could also be evaluated with H2DCFDA staining method as seen in Figure 3 (c). Figure 3 (d) histogram intensity showed the intracellular ROS level was significantly higher in the combination of CUR and AF than in individual AF or CUR.
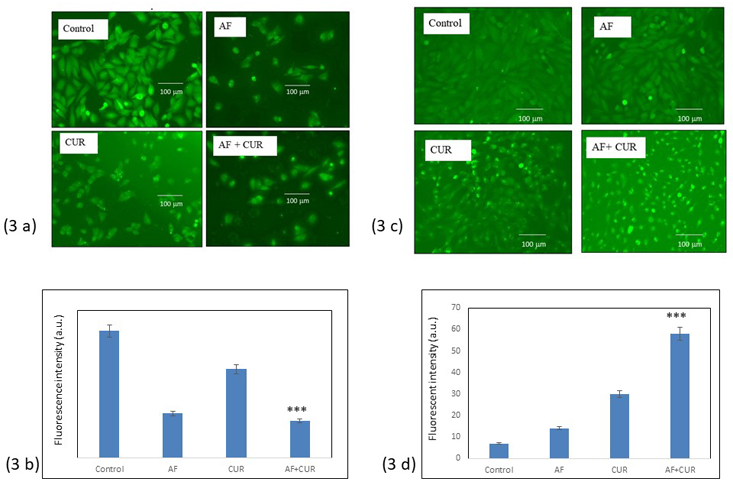
Figure 3: (a) Fluorescence microscopic result from changed in mitochondrial membrane potential after staining with Rhodamine 123. Mitochondrial membrane depolarization was observed in all treated cells as compared to control Saos-2 cells. The Rhodamine123 fluorescence intensity, i.e. mitochondrial membrane potential decreased were more pronounced in combinational treatment than individual AF or CUR. Scale bar indicates 100μm. 3 (b) Histogram showed Significant (*** = 0.001< p) decreased in fluorescence intensity of combination AF and CUR than control. 3 (c) Fluorescence microscopic images of intracellular reactive oxygen species (ROS) generated in Saos-2 cells after 24 h treatment with AF, CUR or their combination using H2DCFDA staining method. Combination AF and CUR treatment significantly had higher levels of ROS than control, individual AF or CUR. Scale bar indicates 100μm (*** = 0.001< p). 3 (d) Histogram showed the relative intensities of Saos-2 cell fluorescence from different groups.
CUR and AF combination treatment increased cell death
The number of cell death in Saos-2 cells treated with individual AF, CUR, or their combination were evaluated with nuclear-ID red/green cell viability as seen in Figure 4 (a). Showed the red fluorescent indicated cells were alive permeable to nucleic acid dye while green fluorescent indicated cells were death impermeable to nucleic acid dye. When the image from red dye and green dye were merged, cells that were alive had red or dark orange color, as seen in the control. Combination of AF and CUR showed the highest number of cell deaths as seen in merge photograph showing green or yellow color. Figure 4 (b) histogram showed the number of cell death was significantly higher in the combination treatment than individual AF or CUR treatment.
The number of cell death in Saos-2 cells treated with individual AF, CUR, or their combination were evaluated with nuclear-ID red/green cell viability as seen in Figure 4 (a). Showed the red fluorescent indicated cells were alive permeable to nucleic acid dye while green fluorescent indicated cells were death impermeable to nucleic acid dye. When the image from red dye and green dye were merged, cells that were alive had red or dark orange color, as seen in the control. Combination of AF and CUR showed the highest number of cell deaths as seen in merge photograph showing green or yellow color. Figure 4 (b) histogram showed the number of cell death was significantly higher in the combination treatment than individual AF or CUR treatment.
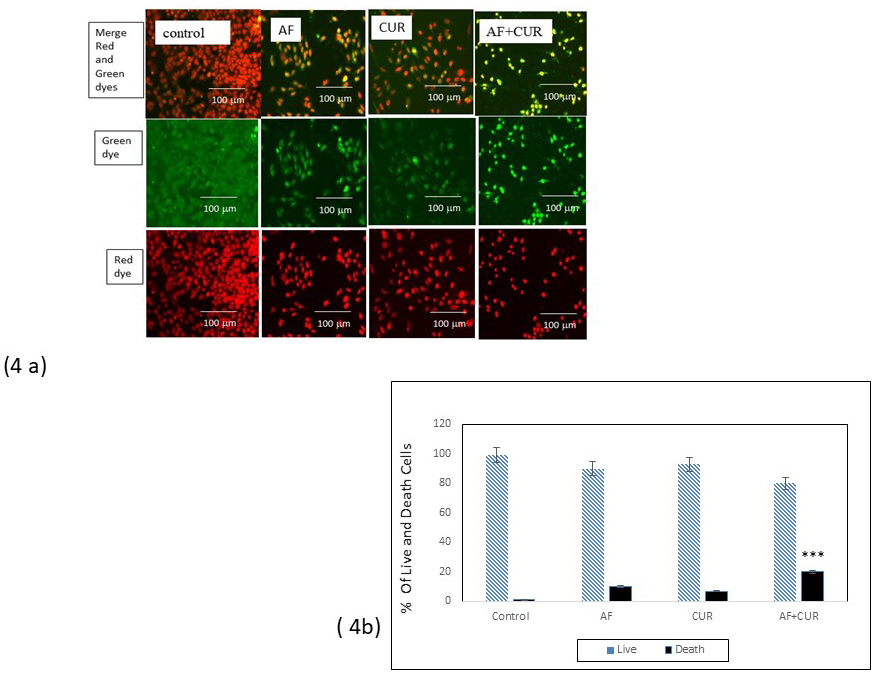
Figure 4: (a) Fluorescence microscopic result from alive and death nucleic acid staining using nuclear-ID red/ green cell viability method. The red fluorescent indicated cells were alive permeable to nucleic acid dye, while green fluorescent indicated cells were death impermeable to nucleic acid dye. When the image from red and green dye were merged, cells that were alive had red or dark orange color. Individual AF or CUR treatment significantly had less cells death than the combination of AF and CUR that showed green or yellow color. Scale bar indicates 100μm. 4 (b) Histogram showed Significant decreased in fluorescence intensity of combination AF and CUR than control (*** = 0.001< p).
CUR and AF combination treatment modulated Bax, Bcl2 and cytochrome C expressions
Intracellular ROS generation in apoptotic cells was associated with change in Bax and Bcl2 protein expressions. Western blot analysis data as seen in Figure 5 (a) and band intensity histogram (Figure 5 b) showed a significant increase in Bax expression and decreased in Bcl2 expressions in Saos-2 cells treated with combination of CUR and AF than its individual CUR or AF. ROS generation also caused reduction in mitochondrial membrane potential that caused increased in the release of cytochrome C into cytosol that further induces apoptosis. Combination of CUR and AF significantly increased expression of cytochrome C
Intracellular ROS generation in apoptotic cells was associated with change in Bax and Bcl2 protein expressions. Western blot analysis data as seen in Figure 5 (a) and band intensity histogram (Figure 5 b) showed a significant increase in Bax expression and decreased in Bcl2 expressions in Saos-2 cells treated with combination of CUR and AF than its individual CUR or AF. ROS generation also caused reduction in mitochondrial membrane potential that caused increased in the release of cytochrome C into cytosol that further induces apoptosis. Combination of CUR and AF significantly increased expression of cytochrome C
CUR and AF combination treatment modulated mitochondrial signaling proteins
Mitochondrial apoptosis due to the depletion of mitochondrial membrane potential had been associated with several key proteins such as Casp 9, Casp 3 and Apaf-1. Western blot analysis showed expression of Apaf-1 was increased in individual CUR or AF as compared to the control. But when the cells were exposed to the combination treatment of CUR and AF, the expression of Apaf-1 increased significantly as seen in Figure 5(c). Casp 3, Casp 9, and PARP protein cleaved to its active form considered as a very important event in cancer cell apoptosis. The expression of Cl. Casp 3 was higher in combination of CUR and AF. Cleavage of PARP and higher expression of Cl. PARP in the combination CUR and AF assured that the apoptosis induced by the combination treatment involved inter-nucleosomal DNA fragmentation related to PARP cleavage. The band intensity histogram seen in Figure 5 (d) showed significant increase of Apaf-1, Casp 9, Cl. Casp 3 and Cl. PARP in CUR and AF combination than individual treatments.
Mitochondrial apoptosis due to the depletion of mitochondrial membrane potential had been associated with several key proteins such as Casp 9, Casp 3 and Apaf-1. Western blot analysis showed expression of Apaf-1 was increased in individual CUR or AF as compared to the control. But when the cells were exposed to the combination treatment of CUR and AF, the expression of Apaf-1 increased significantly as seen in Figure 5(c). Casp 3, Casp 9, and PARP protein cleaved to its active form considered as a very important event in cancer cell apoptosis. The expression of Cl. Casp 3 was higher in combination of CUR and AF. Cleavage of PARP and higher expression of Cl. PARP in the combination CUR and AF assured that the apoptosis induced by the combination treatment involved inter-nucleosomal DNA fragmentation related to PARP cleavage. The band intensity histogram seen in Figure 5 (d) showed significant increase of Apaf-1, Casp 9, Cl. Casp 3 and Cl. PARP in CUR and AF combination than individual treatments.
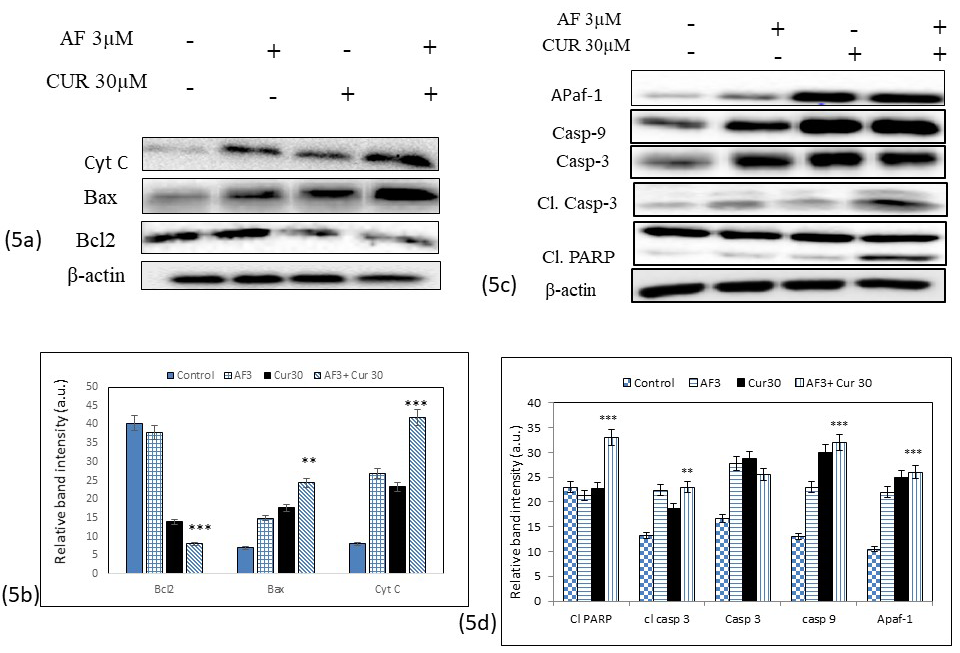
Figure 5: (a) Western blot analysis data showed modulation expressions of Bax, Bcl2 and cytochrome C proteins after treatment with individual CUR, AF or their combinations. Bax expression was significantly (** = 0.001< p) higher in combination treatment than individual CUR or AF treatment., while expression of Bcl2 decreased significantly (*** = 0.01< p) in combination treatment. Cytochrome c expression were higher in individual CUR or AF treatment with the highest expression was noticed in the combination of AF and CUR. 5 (b) Histogram showed ban intensity from the expression of Bax, Bcl2, and Cytochrome c proteins. 5 (c) Western blot analysis of different mitochondrial signaling proteins in Saos-2 cells. Activity of Cl. PARP, Apaf-1, Casp 9, Casp 3, and Cl. Casp 3 were significantly up-regulated in the combination treatment of CUR and AF as compared to the control (*** = 0.001< p). 5 (d) Histogram represents up-regulation of Apaf-1, Cl. PARP, Casp 3 and Casp 9 in the combination over individual CUR or AF treatment.
CUR and AF combination treatment inhibited cell cycle regulatory proteins
Inhibition of cell cycle progression is also considered as one of an important strategy in the management of osteosarcoma. Western blot analysis data seen in Figure 6 (a) and the band intensity histogram Figure 6 (b) showed that the expressions of CDK7, CDK2, cyclin D1 and cyclin B1 were significantly decreased in Saos-2 cells treated with combination of CUR and AF than individual CUR or AF treatments.
Inhibition of cell cycle progression is also considered as one of an important strategy in the management of osteosarcoma. Western blot analysis data seen in Figure 6 (a) and the band intensity histogram Figure 6 (b) showed that the expressions of CDK7, CDK2, cyclin D1 and cyclin B1 were significantly decreased in Saos-2 cells treated with combination of CUR and AF than individual CUR or AF treatments.
CUR and AF combination treatment inhibited matrix metalloproteinase (MMPs)
To determine the potential mechanism of how combination of CUR and AF causing inhibition of cells migration, the invasion associated MMP2 and MMP9 proteins expression was analyzed as determined by Western blot analysis Figure 6(c). Band intensity histogram Figure 20 showed the expression of MMP2 and MMP9 were significantly decreased when SaoS-2 cells were treated with CUR only or combination of CUR and AF. Unchanged MMP9 level and increased in MMP2 expression in cells treated with AF only significantly showed that CUR had synergistic effect with AF on MMP2 and MMP9 expression in Saos-2 cells as seen in Figure 6 (d).
To determine the potential mechanism of how combination of CUR and AF causing inhibition of cells migration, the invasion associated MMP2 and MMP9 proteins expression was analyzed as determined by Western blot analysis Figure 6(c). Band intensity histogram Figure 20 showed the expression of MMP2 and MMP9 were significantly decreased when SaoS-2 cells were treated with CUR only or combination of CUR and AF. Unchanged MMP9 level and increased in MMP2 expression in cells treated with AF only significantly showed that CUR had synergistic effect with AF on MMP2 and MMP9 expression in Saos-2 cells as seen in Figure 6 (d).
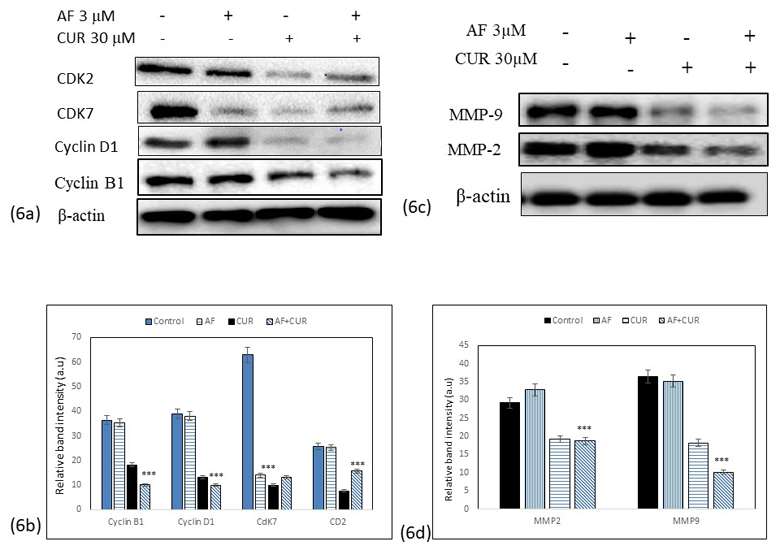
Figure 6: (a) Western blot analysis of proteins involved in cell cycle regulation such as CDK7, CDK2, Cyclin D1 and Cyclin B1 after treatment of Saos-2 cells with individual CUR, AF or their combinations. The expressions of these proteins were down-regulated in all treatment groups. The combination treatment showed more downregulation of proteins than individual CUR or AF treatment. 6 (b) Histogram showed the significant decrease of band intensity in all proteins being expressed (*** = 0.001< p). 6 (c) Western blot analysis of expression of MMP2 and MMP9 in Saos-2 cells treated with individual CUR, AF or their combinations. (d) Histogram represents down-regulation of MMP2 and MMP9 in the combination treatment was significantly lower than individual CUR or AF treatment (*** = 0.001< p).
CUR and AF combination treatment inhibited MEK kinase pathway
To determine the potential mechanism of how combination of CUR and AF involved in aberrant cell signaling of mitogen –activated protein kinase (MAPKs), the active and phosphorylate form mitogen-activated protein kinases kinase (MEK) were analyzed by Western blot analysis. Significant decreased in MEK -1, -2, -3 and -4 expressions were identified in combination treatment of CUR and AF as seen in Figure 7 (a), with band intensity histogram depicted (Figure 7 b). Addition of CUR to AF potentiated its effect in causing decreased in MEK -1, -2, -3 and -4 expressions further.
To determine the potential mechanism of how combination of CUR and AF involved in aberrant cell signaling of mitogen –activated protein kinase (MAPKs), the active and phosphorylate form mitogen-activated protein kinases kinase (MEK) were analyzed by Western blot analysis. Significant decreased in MEK -1, -2, -3 and -4 expressions were identified in combination treatment of CUR and AF as seen in Figure 7 (a), with band intensity histogram depicted (Figure 7 b). Addition of CUR to AF potentiated its effect in causing decreased in MEK -1, -2, -3 and -4 expressions further.
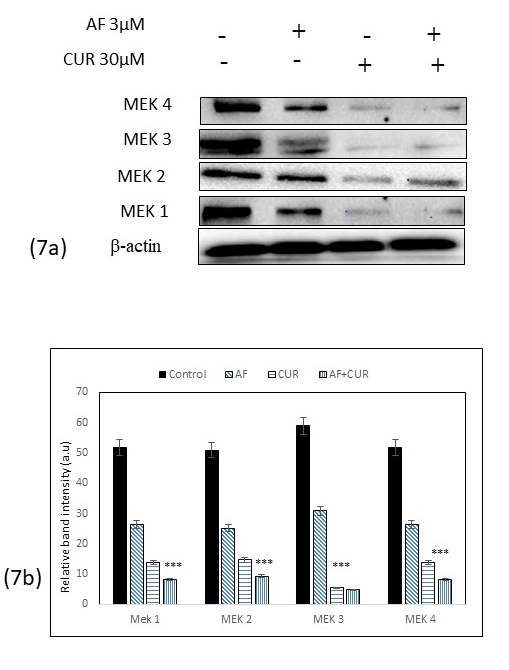
Figure 7: (a) Western blot analysis of MEK-1, -2, -3, and -4 after treatment of Saos-2 cells with individual CUR, AF or their combinations. The expressions of these proteins were down-regulated in all treatment groups. However, in the combination treatment, more down-regulation of MEK 1 to MEK 4 proteins were observed than individual CUR or AF treatment. 7 (b) Histogram showed the significant decrease of band intensity in all these proteins (*** = 0.001< p).
Discussion
In the present study, we have provided convincing evidence that CUR in combination with AF enhanced the anti-proliferative and pro-apoptotic effects in vitro of OS cells by allowing use of lower dose of AF as an anti-cancer agent. Although the two compounds have each been extensively studied, to the best of our knowledge this study is the first to show that combining CUR with AF provided benefit synergistic effect in Saos-2 cells.
Chemotherapeutic drugs are usually not given as monotherapy to prevent drug resistant, but mostly administered with other chemotherapeutics or non-chemotherapeutic agents to achieve synergistic effect. Combining plant compounds that have anti-cancer properties with conventional chemotherapeutic drug at their lower individual doses can greatly improves the therapeutic outcome of cancer treatments due to their combined action in increasing the apoptotic efficacy or in decreasing resistance with the advantage of causing more tolerable or less side effects to the normal cells [25]. The aim of the present study is to use the lowest possible concentration of AF to decrease undesirable toxicity to normal cells, by combining its effects with high concentration of CUR. In several studies, it had been shown that CUR at high dose of 10 mM- 100 mM has inhibitory effect on different cancers, without causing undesirable side effects to normal cells [2-7]. Clinical trials with CUR also indicated safety, tolerability, efficacy, and non-toxic effect when used as an adjuvant in cancer treatment, for up to oral dose of 8000 mg/day [8].
In this study, the combination of CUR and AF reduced the Saos-2 cells viability to a higher extent than their individual treatments. Hoechst 33342 staining data suggested that the combination of CUR and AF increased the nuclear condensation of the apoptotic cells as compared to the individual AF or CUR treatment. The nuclear-ID red/green cell staining data reaffirmed that more cells death were observed in cells treated with combine AF and CUR.
Evidences suggested that cancer cells generate more intracellular ROS which cause cells to apoptosis more than normal cells [26]. Our present study showed that the combination of CUR and AF significantly caused increase in the ROS levels indicating the important role of ROS in Saos-2 cell apoptosis as confirmed by H2DCFDA staining assay. Increased ROS level in OS cells is associated with the modulation of several key signaling proteins involve in cell cycle regulation and differentiation, tumor suppression, apoptosis, and damage to DNA [27-28]. ROS plays crucial role in the modulation of pro-apoptotic Bax and anti-apoptotic Bcl2. In this study, up regulation of Bax and down regulation of Bcl2 provided an insight that combination of CUR and AF could initiate a favorable signal transduction mechanism to cause cancer cells to apoptosis. In addition, activation of Apaf-1 and Cl. Casp 9 formed apoptosome that further activates downstream executioner Casp 3, the protein that play a central role in the execution of apoptosis and the cleavage of PARP during cell death. In this study, we demonstrated apoptotic cascade initiation through activation of Apaf-1, enhanced expression of Casp 9, Casp 3 and its cleaved form in the combination of CUR and AF group.
Studies also showed Bax/Bcl2 modulation is proceeded by the collapse of mitochondrial membrane potential, which has been associated with the initiation of caspase cascade leading to cell death [29]. The present study showed that the combination of CUR and AF significantly decreases the mitochondrial membrane potential, indicating a causative role of ROS in mitochondrial dysregulation. Reduction in mitochondrial membrane potential also leads to the release of cytochrome c and other apoptosis-inducing factor into cytosol which further induces apoptosis events [30-31].
Proliferation of several cancers are also largely associated with altered in the regulation of cell cycle; therefore, targeting cell cycle proteins in cancer chemotherapy provide a promising future [32]. Distinct phases of the cell cycle (G0/G1, S, G2 and M) are regulated by several cyclin-dependent kinases that bind to a specific regulatory subunit cyclin (CDKs) [32]. Among the cyclins, cyclin D1 together with cyclin C and – E inhibit G1 phase, while cyclin B1 inhibits G2/M phase, CDK2 inhibit G1/S phase, and CDK7 inhibit G2 phase, respectively [32-34]. In our study, significant down regulation cyclin D1, cyclin B1, CDK2 and CDK7 strongly suggest that combination of CUR and AF effectively inhibited OS at different phases of cell cycle as compared to control cells
Another important strategy as cancer chemotherapeutic agents are aimed to prevent angiogenesis. Matric degrading enzymes such as matrix metallo proteinase (MMP)-2 and -9 play crucial role in most cancer metastasis including OS [35-36]. Significant decreased in the MMP-2 and MMP-9 expression suggested that combination of CUR and AF effectively inhibited invasion and metastasis of OS cells.
MAPKs are Ser/Thr kinases that convert extracellular stimuli into a wide range of cellular responses such as proliferation, differentiation, development, transformation, and apoptosis [37-38]. Combination of CUR and AF caused decreased in MEK -1, -2, -3 and -4 expressions. These proteins have been expressed in more progressive cancer; therefore, chemotherapeutic agent that targeted this pathway provide promising future [39-41].
The results of our study suggest that CUR synergistically work with AF in human OS therapy in causing cell death as indicated by the accumulation of intracellular ROS, by activation of proteins involved in mitochondrial signaling pathway, by inhibition of migration, by inhibition of cell cycle progression, and by inhibition of angiogenesis. This study may have a useful implication for potential application of CUR as a chemo-enhancing neo-adjuvant with AF in human OS therapy by achieving higher efficacy and potency with minimum side effects.
Conclusion
Our findings was the first to suggest that CUR, a natural antioxidant, synergistically work with anti-cancer AF to inhibit cell growth and to cause cell death in human osteosarcoma Saos-2 cells. Combination of low dose anticancer AF and antioxidant CUR worked synergistically by causing induction of pro-apoptotic proteins such as apaf-1, casp 9, casp 3 and Bax and by inhibition of proteins that can cause cancer cells become resistant such as of BCl2, cyclin D1, CDK2, CDK7 and cyclin B1, and MEK-1 to -4. This preliminary study merits further investigation with animal studies and possible clinical trials in humans. Our study was also the first to indicate use of AF in osteosarcoma treatment.
Acknowledgments
The authors thank Union University for providing funding for this research
ORCID ID : Lunawati Bennett ID https://orcid.org/0000-0002-2193-8837
The authors thank Union University for providing funding for this research
ORCID ID : Lunawati Bennett ID https://orcid.org/0000-0002-2193-8837
Copyright
This work has not been published before and it is not under consideration for publication elsewhere.
This work has not been published before and it is not under consideration for publication elsewhere.
References
- Eilber BF, Guiliano A, Eckardt J. (1987). Adjuvant chemotherapy for osteosarcoma: a randomized prospective trial. J Clin Oncol 5: 21-26.
- Shakibaei M, Kraehe P, Popper B, Shayan P, Goel A, Buhrmann C (2015). Curcumin potentiates antitumor activity of 5-fluorouracil in a 3D alginate tumor microenvironment of colorectal cancer. BMC Cancer. 15: 250.
- Hao F, Kang J, Cao Y, Fan S, Yang H, An Y (2015). Curcumin attenuates palmitate-induced apoptosis in MIN6 pancreatic β-cells through PI3K/Akt/FoxO1 and mitochondrial survival pathways. Apoptosis. 20: 1420–1432.
- Zhou Q, Ye M, Lu Y, Zhang H, Chen Q, Huang S (2015). Curcumin improves the tumoricidal effect of mitomycin C by suppressing ABCG2 expression in stem cell-like breast cancer cells. PLoS One. 10. e0136694.
- Land B, Blijlevens NM , Marteijn J, Timal S, Donnelly JP, Rabet LM (2004). Role of curcumin and the inhibition of NF-kappa B in the onset of chemotherapy-induced mucosal barrier injury. Leukemia. 18:276–284.
- Yosifov DY, Kaloyanov KA, Guenova ML, Prisadashka K, Balabanova MB, Konstantinov SM (2014). Alkylphosphocholines and curcumin induce programmed cell death in cutaneous T-cell lymphoma cell lines. Leuk Res. 38: 49–56.
- Zhou QM, Wang XF, Liu XJ, Zhang H, Lu YT , Huang S (2011). Curcumin improves MMC-based chemotherapy by simultaneously sensitizing cancer cells to MMC and reducing MMC-associated side-effects. Eur J Cancer. 47: 2240–2247.
- Shanmugam MK , Rane G , Kanchi MM, Arfuso F, Chinnathambi A, Zayed ME (2015). The multifaceted role of curcumin in cancer prevention and treatment. Molecules. 20 :2728–2769.
- Zhang Y, Chen P, Hong H, Wang L, Zhou Y, Lang Y (2017). JNK pathway mediates curcumin-induced apoptosis and autophagy in osteosarcoma MG63 cells. Exp Ther Med. 14: 593–599.
- Teiten MH, Eifes S, Dicato SM, Diederich M (2010). Curcumin: The Paradigm of a Multi-Target Natural Compound with Applications in Cancer Prevention and Treatment. Toxins (Basel) 2: 128-162.
- Kager L, Zoubek A, Potschger U (2003). Primary metastatic osteosarcoma: presentation and outcome of patients treated on neoadjuvant cooperative osteosarcoma study group protocols. J Clin Oncol 21: 2011-2018.
- Pakos EE, Nearchou AD, Grimeret RJ (2009). Prognostic factors and outcomes for osteosarcoma: an international collaboration. Eur J Cancer 45: 2367-2375.
- Cho WH, Song WS, Jeon DG (2010). Differential presentation, clinical courses and survivals of osteosarcomas of the proximal humerus over the extremity locations. Ann Surg Oncol 17: 702-708.
- Amirhossein A, Goldin M, Awad M Kulidjian A (2018). Osteosarcoma: a comprehensive review. SICOT J 4 :1-12.
- https://seer.cancer.gov/statfacts/html/bones.html. Access May 20, 2021.
- Mirabello L, Troisi RJ, Aavage SA (2009). International osteosarcoma incidence patterns in children and adolescent, middle ages and elderly persons. Int J Cancer 125: 229-234.
- Hurvitz SA, Shatsky R, Harbeck N (2014). Afatinib in the treatment of breast cancer. Expert Opin Investig Drugs. 23:1039–1047.
- Molife LR, Omlin O, Jones RJ, Karavasilis V, Bloomfield D, Lumsden G (2014). Randomized Phase II trial of nintedanib, afatinib and sequential combination in castration-resistant prostate cancer. Future Onc 10: 219–231.
- Seiwert TY , Fayette J , Cupissol D, Del Campo JM, Clement PM, Hitt R (2014). A randomized, phase II study of afatinib versus cetuximab in metastatic or recurrent squamous cell carcinoma of the head and neck. Ann of Oncol ESMO. 25: 1813–1820.
- Wang S, Liu S , Zhao B, Yang F, Wang Y , Liang Q (2015). Afatinib reverses multidrug resistance in ovarian cancer via dually inhibiting ATP binding cassette subfamily B member 1. Oncotarget.6: 26142–26160.
- Li D, Ambrogio L, Shimamura T (2008). BIBW2992, an irreversible EGFR/HER2 inhibitor highly effective in preclinical lung cancer models. Oncogene 27:4702-4711.
- Solca F, Dahl C, Zoephel A (2012). Target binding properties and cellular activity of afatinib (BIBW 2992), an irreversible ErbB family blocker. J Pharmacol Exp Ther. 343: 342-350.
- Dong L, Lei D, Zhang H (2017). Clinical strategies for acquired epidermal growth factor receptor tyrosine kinase inhibitor resistance in non-small-cell lung cancer patients. Oncotarget. 8: 64600–64606.
- Mondal A, Bennett LL (2016). Resveratrol enhances the efficacy of sorafenib mediated apoptosis in human breast cancer MCF7 cells through ROS, cell cycle inhibition, caspase 3 and PARP cleavage. Biomed Pharmacother 84:1906-1914.
- Kumar D, Basu S, Parija L, Rout D, Manna S, Dandapat J (2016). Curcumin and ellagic acid synergistically induce ROS generation, DNA damage, p53 accumulation and apoptosis in HeLa cervical carcinoma cells. Biomed. Pharmacother. 81: 31-37.
- Simon HU, Haj-Yehia A, Levi-Schaffer F (2000). Role of reactive oxygen species (ROS) in apoptosis induction. Apoptosis. 5: 415-418.
- Mates JM, Sanchez-Jimenez FM (2000). Role of reactive oxygen species in apoptosis: implications for cancer therapy. Int. J. Biochem. Cell Biol. 32 : 157-170.
- Zhang T, Brazhnik P, Tyson JJ (2007). Exploring mechanisms of the DNA-damage response: p53 pulses and their possible relevance to apoptosis. Cell Cycle. 6: 85-94.
- Penninger JM, Kroemer G (2003). Mitochondria, AIF and caspases-rivaling for cell death. Nature Cell Biol. 5: 97-99.
- Jin S, Xu HG, Shen JN, Chen XW, Wang H, Zhou JG (2009). Apoptotic effects of curcumin on human osteosarcoma U2OS cells. Ortho Surg 1: 144-152.
- Yang Y, Liu X, Bhalla K (1997). Prevention of apotosis by Bcl-2 release cytochrome c from mitochondria blocked. Science 275: 1129-1132.
- Otto T, Sicinski P (2017). Cell cycle proteins as promising targets in cancer therapy. Nat Rev Cancer 17: 93-115.
- Choi YJ, LI A, Hydbring P, Sandam T, Stefano J, Christie AL (2012). The requirement for cyclin D1 function in tumor maintenance. Cancer Cell 22: 438-451.
- Okuda M, Horn HF, Tarapore P, Tokuyama Y, Smulian SG, Chan PK (2000). Nucleophasmin/B23 is a target of CDk2/cyclin E in centrosome duplication. Cell 103: 127-140.
- Posthumadeboer J, Witlox MA, Kaspers GJ, van Royen BJ (2011). Molecular alterations as target for therapy in metastatic osteosarcoma: A review of literature. Clin Exp Metast 28 (5): 493-503.
- Ando K, Mori K, Verrecchia F, Marc B (2012). Molecular alterations associated with osteosarcoma development. Sarcoma 523432.
- Comsa S, Cimpean AM, Raica M (2015). The story of MCF-7 breast cancer cell line: 40 years of experience in research. Anticancer Res 35: 3147-3154.
- Ryu M, Sung CK, Im YJ, Chun CJ (2020). Activation of JNK and p38 in MCF-7 cells and in vitro anticancer activity of alnus histula extract. Molecules 25(5): 1073.
- Yin G, Fan J, Zhou W, Ding Q, Zhang J, Wu X (2017). ERK inhibition sensitizes CZ415-induced anti-osteosarcoma activity in vitro and in vivo. Oncotarget 8: 82027-82036.
- Li Y, Dong Q, Cui Y (2019). Synergistic inhibition of MEK and reciprocal feedback networks for targeted intervention in malignancy. Cancer Bio Med 16: 415-434.
- McCubrey JA, Steelman LS, Chappell WH, Abrams SL, Wong EW, Chang F (2007). Roles of the RAF/MEK/ERK pathway in cell growth, malignant transformation and drug resistance. Biochim Biophys Acta 1773: 1263-1284.
Citation: Lunawati L Bennett and Arindam Mondal. (2021). Curcumin and Afatinib Synergistically Inhibit Growth of Human Osteosarcoma Cells by Inhibition of Matrix Metallo Proteinases, Mitogen Activated kinases 1-4, and Reactive Oxygen Species. Journal of Pharmacy and Drug Development 3(1).
DOI: 10.5281/zenodo.5125049
DOI: 10.5281/zenodo.5125049
Copyright: © 2021 Lunawati L Bennett. This is an open-access article distributed under the terms of the Creative Commons Attribution License, which permits unrestricted use, distribution, and reproduction in any medium, provided the original author and source are credited.