Review Article
Volume 6 Issue 1 - 2024
Stem Cell Therapies for Chronic Wound Management: A Comprehensive Review
1MD, PhD, Cancer Biology and Genetics Program, Sloan Kettering Institute, Memorial Sloan Kettering Cancer Center, New York, NY 10065, USA
2MD, MS, Weill Cornell Medical College of Cornell University
3MD, MS, Nexus Alliance Biopharma
4PharmD, Nexus Alliance Biopharma
5Student at the University of Florida
6Student at York University, Toronto, ON
7Student at Valencia College
2MD, MS, Weill Cornell Medical College of Cornell University
3MD, MS, Nexus Alliance Biopharma
4PharmD, Nexus Alliance Biopharma
5Student at the University of Florida
6Student at York University, Toronto, ON
7Student at Valencia College
*Corresponding Author: Timothy Allen, MD, PhD, Cancer Biology and Genetics Program, Sloan Kettering Institute, Memorial Sloan Kettering Cancer Center, New York, NY 10065, USA.
Received: October 03, 2024; Published: October 10, 2024
Abstract
Chronic wounds, particularly prevalent among patients with diabetes and peripheral arterial disease, pose significant health challenges and financial burdens, with treatment costs exceeding $22 billion annually. Conventional treatment methods, including autografts and debridement, often involve painful procedures and limited efficacy. Stem cell therapies have emerged as a promising alternative, offering potential improvements in wound healing, reduced morbidity, and lower amputation rates. This review examines the therapeutic potential of various stem cell sources, including placental-derived mesenchymal stem cells, bone marrow-derived mesenchymal stem cells (BM-MSCs), induced pluripotent stem cells (iPSCs), Wharton's Jelly stem cells, and adipose-derived stem cells (ADSCs). Key findings demonstrate that stem cells can accelerate wound closure, enhance paracrine signaling, and promote angiogenesis, particularly in diabetic wound models. However, challenges remain, such as the variability in stem cell sources, potential immune reactions, and limitations in current clinical trials. Despite these challenges, the overall trend indicates significant potential for stem cell therapies in chronic wound management. Further research, including larger, blinded, randomized controlled trials, is necessary to optimize treatment protocols and confirm the efficacy of these innovative therapies
Keywords: Chronic wounds, stem cell therapy; Mesenchymal stem cells; Induced pluripotent stem cells; Adipose-derived stem cells
Article
Chronic wounds are a devastating complication that may result in amputation and reduced quality of life. The estimated cost burden to society is 22 billion dollars each year [1]. Chronic wounds often occur in patients who have diabetes or peripheral arterial disease [1]. Current treatment relies on allografts from the patient to retrieve healthy skin, debridement, and cleaning the wound to help it heal [1]. Obtaining the allograft can be painful and traumatic. The use of stem cells to treat and heal wounds shows promise in preventing morbidity and mortality. Scientists are investigating ways to engineer stem cells to help the patient’s body elicit the proper channels to heal the wound.
Stem cells obtained from sick and/or elderly individuals may not be as robust, able to differentiate or exist in sufficient quantity to perform adequately in medical settings. Also, extracting stem cells from bone marrow is painful and may result in infection or pain. Fortunately, stem cells derived from the placenta have been shown to perform as well as stem cells obtained from an adult [2]. The investigators compared mesenchymal stem cells obtained from an adult and those obtained from a placenta between 38-40 weeks gestation. Stem cells obtained from the placenta are in a much higher concentration than compared to the bone marrow or adipose tissue [2]. Placental stem cells have less epigenetic imprinting and are easier to differentiate [2]. Cell surface markers in placental-derived stem cells have shown to be similar to bone marrow-derived mesenchymal stem cells2. Placental-derived mesenchymal stem cells demonstrate performance comparable to their adult-derived counterparts in tissue regeneration and repair [2]. This similarity in function underscores the potential of the placenta as a valuable reservoir of stem cells for therapeutic applications. These cells possess the capacity to differentiate into various types of endothelial cells, offering significant promise in wound healing therapies [2].
Although the placenta is a reservoir of stem cells, bone marrow-derived mesenchymal stem cells (BM-MSC) are often still used and more readily available. Paracrine factors from BM-MSC have been shown to accelerate the rate of healing of wounds in a murine model [3]. Chen and colleagues compared BM-MSC to dermal fibroblasts in their ability to heal wounds in a murine model. According to the authors, paracrine factors (IGF-1, EGF, KGF, VEGF-α) from the BM-MSC in a conditioned medium accelerated wound closure compared to dermal fibroblasts [3]. The paracrine factors may accelerate wound closure by enhancing the migration of macrophages, endothelial cells, and keratinocytes. Paracrine factors from BM-MSCs in a conditioned medium may offer hope to patients with chronic wounds. While bone marrow-derived mesenchymal stem cells (BM-MSCs) remain a common choice in stem cell therapy, the discovery of induced pluripotent stem cells (iPSCs) has significantly expanded the therapeutic options available to researchers and clinicians in addressing chronic wound management. Adult stem cells or embryonic stem cells may not always be available for a patient. Allogenic stem cells may cause an immune reaction due to an HLA mismatch while iPSC mitigate the risk of any immune reaction because they can be autologous. Induced pluripotent stem cells are able to help heal wounds because they secrete angiogenic factors, increase paracrine signaling, and they incorporate themselves into the vasculature of the wound [4]. Induced pluripotent stem cells may serve as a viable source of stem cells in the near future for chronic wound treatment. Advantages and disadvantages of different stem cell modalities are highlighted in Figure 2.
Many wounds become chronic because the patient has diabetes. Diabetes impairs the ability of wounds to heal and stem cell treatment may offer a method of helping patients. Embryonic stem cell extracts (EXT) demonstrated enhanced epithelialization and accelerated wound healing in diabetic mice compared to fibroblast extracts and saline controls [5]. Stem cells have demonstrated that they can help heal chronic wounds in a murine model; however, outcomes in mice often don’t translate over to humans. Fortunately, there is an abundance of data that demonstrates that stem cells have the ability to help heal wounds in humans as well.
Wharton’s Jelly stem cells have often been used by regenerative medicine specialists to help patients with a variety of diseases. Stem cells are often placed on scaffolds, which serve as a place for the cells to grow and integrate into the tissue. Wharton’s Jelly stem cells are easier to obtain than mesenchymal stem cells and have a higher proliferation capacity6. Wharton’s Jelly stem cells have demonstrated the ability to differentiate into fibroblast, epithelial, and endothelial cells, which are all essential for any wound to heal [6]. The trial had patients that ranged in age from 30-60 years old. The trial may not be applicable as most patients with diabetic wounds are quite elderly. The wounds were treated with Wharton’s Jelly stem cells on a scaffold every 3 days for 9 days. Mean wound size decreased from 70.96mm2 before treatment to 3.07mm2 over one month [6]. The patients experienced a significant decrease in wound size and decrease in pain. This may be because Wharton’s Jelly secreted growth factors, prevented infection, and promoted angiogenesis6. Stem cells applied to a wound in combination with a scaffold might serve as a means to help heal chronic diabetic wounds.
Bone marrow-derived autologous mesenchymal stem cells (BMMSC), while not having the ability to differentiate as easily as stem cells derived from the umbilical cord, have a lower risk of immune rejection. Patients ineligible for surgical revascularization may benefit from stem cell therapies as an alternative treatment. A trial was conducted to test the efficacy, feasibility, and safety of autologous bone marrow-derived stem cells (BMMSC). They were compared to expanded bone marrow cells (TRC) and placebo to treat diabetic foot ulcers. Both intervention groups showed improvements in wound healing and most patients avoided amputation [7]. The study was not powered to detect differences between the groups and many patients in the placebo group dropped out. The study was also limited by the small sample size and lack of blinding. Larger placebo-controlled trials are needed to confirm the efficacy of the stem cell product.
Placental Derived Stem Cells | Induced Pluripotent Stem Cells | Adult Stem Cells | |
Advantages | Pluripotent-Able to differentiate into almost any tissue No ethical concerns Easy to culture and isolate |
Somewhat pluripotent No ethical concerns Less concern for immune rejections |
Readily available No ethical concerns Less concerns for immune rejections |
Disadvantages | Tissue rejection risks Not always readily available Possibility of teratoma formation |
Limited differentiation capacity Difficult to differentiate Prone to genetic abnormalities |
Limited differentiation capacity Not as durable Often painful to obtain |
Figure 1: Advantages and disadvantages of different stem cell sources.
A three-year trial compared autologous bone marrow mesenchymal stem cells (BMMSC), bone marrow-derived mononuclear cells (BMMNC), and placebo in 41 patients with diabetic foot ulcers or limb ischemia. Mononuclear cells are also known as white blood cells [8]. Patients received BMMSC or BMMNC in one limb and saline in the other, serving as their own controls. Both BMMSC and BMMNC-treated limbs showed improved outcomes compared to placebo, with BMMSC demonstrating superior results in amputation rates, pain-free walking time, and angiogenesis at 3-6 months post-injection. After three years, amputation rates were comparable across groups. Further research is warranted to investigate whether periodic BMMSC injections could yield sustained improvements in limb health and reduce long-term amputation rates.
Another method of obtaining autologous stem cells is to administer granulocyte colony-stimulating factor (G-CSF) to elicit the mobilization of hematopoietic stem cells (HSCs) in a patient. This is less painful than most methods of autologous stem cell extraction [9]. Hematopoietic stem cells have the capability to differentiate into endothelial cells in anoxic environments, such as diabetic foot ulcers [9]. In a trial patients were injected with filgrastim, which is a G-CSF, to start HSCs mobilization and then had their venous blood extracted and the HSCs separated. The separated HSCs were then injected into the patients’ wounds. Within 4 weeks after injection, the patients’ claudication symptoms improved, their pain decreased, and they were able to walk farther. New blood vessels formed and skin temperature increased which is an indicator of tissue health and proper blood flow [9]. The method of mobilizing stem cells through the use of G-CSF has the potential to help patients and spare them the pain of having to aspirate stem cells from the iliac crest or adipose tissue.
Not all stem cell mobilization methods prove effective, and some may even impede healing. A study investigated the use of Plerixafor, a CXCR4 antagonist capable of mobilizing hematopoietic stem cells (HSCs) from bone marrow, in an attempt to promote wound healing. However, the results were not as anticipated. [10]. A trial of diabetic patients with chronic wounds was randomized, double blinded and had a placebo group. The mean age was 69 years old and 84.6% were male. This sample demographic closely aligns with the characteristics of the broader population afflicted with chronic, non-healing wounds, particularly those associated with diabetes and other underlying conditions. The trial was actually terminated early because wounds in the group that received Plerixafor had their wounds healed at a much lower rate than the placebo group. No difference was seen in amputation rates. The mobilization of the HSCs by Plerixafor resulted in impaired healing. Further study and investigation might reveal the reasons why Plerixafor failed and potential treatments that may result from that elucidation.
Adipose tissue serves as an alternative source of stem cells for chronic wound treatment [11]. In a study of 16 patients with recalcitrant leg ulcers, half received standard care plus adipose-derived stem cells (ADSC), while the control group received standard care alone. Adipose derived stem cells were isolated and concentrated from harvested adipose tissue via centrifugation. The intervention group demonstrated significantly faster wound healing (17.5 weeks vs. 24.5 weeks) and reduced pain compared to controls, with no major adverse effects reported. Figure 2 illustrates the marked improvement in wound healing for a representative patient from the intervention group. While these results are promising, the study's limitations, including small sample size and lack of blinding, necessitate larger trials to confirm the efficacy of ADSC therapy for chronic wounds.
s
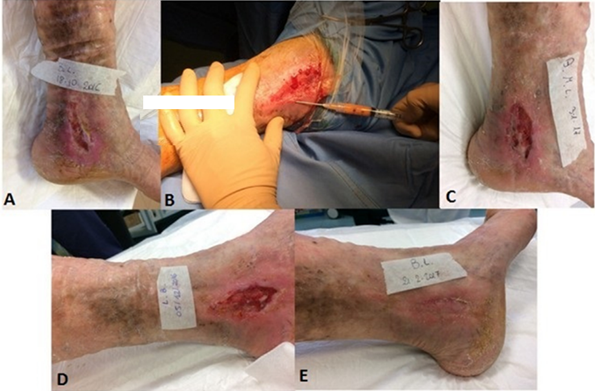
Figure 2: As seen in the pictures below, the patient’s wound healed in a short amount of time after being injected with autologous stem cells derived from adipose tissue. Adapted from Zollino et al.
As seen in the above pictures, the patient’s wound healed in a short amount of time after being injected with autologous stem cells derived from adipose tissue. Adapted from Zollino et al.
The following case studies/clinical trials highlight the medical applications of different stem cell treatments:
A 58-year-old male patient with a chronic diabetic foot ulcer went through a comprehensive foot examination, imaging (X-ray, MRI), and vascular assessment. The prescribed treatment plan involved offloading and debridement of the afflicted foot, followed by treatment with adipose-derived stem cells (ADSCs) applied topically. Further stages of treatment involved systemic antibiotics, advanced wound dressings, and stem cell therapy (using BM-MSCs). This resulted in a significant reduction in wound size and improved healing time [1].
A 72-year-old female patient with a non-healing venous leg ulcer went through a duplex ultrasound, venography, and wound culture. The prescribed treatment plan involved compression therapy and debridement of the afflicted leg, followed by treatment with bone marrow-derived mesenchymal stem cells (BM-MSCs) injected around the wound. Additionally, there was surgical intervention, use of systemic antibiotics, and stem cell therapy (using iPSCs). This resulted in enhanced wound closure and reduced pain [2].
A 65-year-old male patient with a chronic pressure ulcer went through pressure mapping, a wound culture, and nutritional assessment. The prescribed treatment plan involved pressure relief and debridement, as well as incorporating induced pluripotent stem cells (iPSCs) into a hydrogel scaffold. Additional surgical debridement was also required. This resulted in accelerated wound healing and improved tissue regeneration [3].
Clinical trials have show significant improvement in burn wound healing with mesenchymal stem cell (MSC) therapy, including faster healing times and reduced scarring [12]. Comprehensive reviews of clinical trials indicate that MSCs are effective in various wound types, with promising results in both preclinical and clinical settings.
Stem cell transplantation has shown significant promise in enhancing wound healing through various mechanisms. Below is a breakdown of the downstream actions and associated processes:
1. Inflammation Phase
Initial immune modulation occurs, where stem cells (particularly mesenchymal stem cells), modulate the immune response by secreting anti-inflammatory cytokines (including IL-10 and TGF-β) and reducing the infiltration of inflammatory cells [13]. Paracrine signaling also occurs, where growth factors and cytokines are released that help in reducing inflammation and promoting tissue repair [14]. Mesenchymal stem cells (MSCs) interact with various immune cells, including T cells, B cells, and macrophages, to modulate their activity and promote a regenerative environment [15].
Initial immune modulation occurs, where stem cells (particularly mesenchymal stem cells), modulate the immune response by secreting anti-inflammatory cytokines (including IL-10 and TGF-β) and reducing the infiltration of inflammatory cells [13]. Paracrine signaling also occurs, where growth factors and cytokines are released that help in reducing inflammation and promoting tissue repair [14]. Mesenchymal stem cells (MSCs) interact with various immune cells, including T cells, B cells, and macrophages, to modulate their activity and promote a regenerative environment [15].
2. Proliferation Phase
Stem cells promote angiogenesis by secreting pro-angiogenic factors like VEGF (vascular endothelial growth factor) [14]. They also stimulate fibroblasts to produce extracellular matrix components, which are crucial for tissue regeneration [14].
Stem cells promote angiogenesis by secreting pro-angiogenic factors like VEGF (vascular endothelial growth factor) [14]. They also stimulate fibroblasts to produce extracellular matrix components, which are crucial for tissue regeneration [14].
3. Remodeling Phase
Stem cells enhance collagen synthesis and deposition, leading to better wound strength and reduced scarring16. Re-epithelialization also occurs, as stem cells aid in the migration and proliferation of keratinocytes, which are essential for the formation of new skin layers [16].
Stem cells enhance collagen synthesis and deposition, leading to better wound strength and reduced scarring16. Re-epithelialization also occurs, as stem cells aid in the migration and proliferation of keratinocytes, which are essential for the formation of new skin layers [16].
4. Cellular Dynamics
The plasticity of stem cells allows them to differentiate into various cell types needed for tissue repair, including keratinocytes, endothelial cells, and fibroblasts17. Stem cells migrate to the wound site in response to chemotactic signals, ensuring they reach the areas where they are most needed [17]. There, they can secrete growth factors like vascular endothelial growth factor (VEGF), fibroblast growth factor (FGF), and hepatocyte growth factor (HGF) that are crucial for angiogenesis and tissue repair13. Stem cell activity can be identified with surface markers such as CD73, CD90, and CD105 [18].
The plasticity of stem cells allows them to differentiate into various cell types needed for tissue repair, including keratinocytes, endothelial cells, and fibroblasts17. Stem cells migrate to the wound site in response to chemotactic signals, ensuring they reach the areas where they are most needed [17]. There, they can secrete growth factors like vascular endothelial growth factor (VEGF), fibroblast growth factor (FGF), and hepatocyte growth factor (HGF) that are crucial for angiogenesis and tissue repair13. Stem cell activity can be identified with surface markers such as CD73, CD90, and CD105 [18].
5. Treatments
Different types of treatments are used to ensure persistent efficacy of stem cell therapies, including:
Different types of treatments are used to ensure persistent efficacy of stem cell therapies, including:
Combination Therapies: Using MSCs with other treatments like plasma-rich proteins or scaffolds to enhance their efficacy [19].
Biophysical Modalities: Techniques like electrical stimulation and shock wave therapy to promote faster wound regeneration [12].
Engineered Skin Substitutes: Seeding MSCs on scaffolds or matrices to create skin substitutes that can replace damaged tissue [12].
6. Challenges and Considerations
A reliable and effective source of stem cells is required for these processes. Different sources like adipose tissue, bone marrow, and induced pluripotent stem cells (iPSCs) have varying efficacies and challenges [13]. One must also consider the most effective delivery method. These include topical application, scaffold incorporation, and combination with plasma-rich proteins [13]. These mechanisms collectively contribute to improved wound healing outcomes, making stem cell therapy a promising approach in regenerative medicine.
A reliable and effective source of stem cells is required for these processes. Different sources like adipose tissue, bone marrow, and induced pluripotent stem cells (iPSCs) have varying efficacies and challenges [13]. One must also consider the most effective delivery method. These include topical application, scaffold incorporation, and combination with plasma-rich proteins [13]. These mechanisms collectively contribute to improved wound healing outcomes, making stem cell therapy a promising approach in regenerative medicine.
The potential of using stem cell therapies in treating chronic wounds shows great promise across multiple studies and different cell types. Key findings from these studies include accelerated wound healing, reduced pain, and lower amputation rates. There are caveats though, the study using the CXCR4 antagonist demonstrated the complexity in stem cell mobilization. The overall trend is encouraging, but the small sample sizes, lack of blinding, and relatively short follow up periods in many studies underscore the need for larger, blinded, randomized controlled trials. Additionally, the variability in stem cell sources, preparation methods, and delivery techniques across the studies makes it challenging to draw definitive conclusions about what is the optimal approach. The body of research represents a significant step forward but also highlights the critical need for continued clinical trials in this promising area of medicine.
1. Inflammation Phase
Initial immune modulation occurs, where stem cells (particularly mesenchymal stem cells), modulate the immune response by secreting anti-inflammatory cytokines (including IL-10 and TGF-ß) and reducing the infiltration of inflammatory cells [13]. Paracrine signaling also occurs, where growth factors and cytokines are released that help in reducing inflammation and promoting tissue repair [14]. Mesenchymal stem cells (MSCs) interact with various immune cells, including T cells, B cells, and macrophages, to modulate their activity and promote a regenerative environment [15].
Initial immune modulation occurs, where stem cells (particularly mesenchymal stem cells), modulate the immune response by secreting anti-inflammatory cytokines (including IL-10 and TGF-ß) and reducing the infiltration of inflammatory cells [13]. Paracrine signaling also occurs, where growth factors and cytokines are released that help in reducing inflammation and promoting tissue repair [14]. Mesenchymal stem cells (MSCs) interact with various immune cells, including T cells, B cells, and macrophages, to modulate their activity and promote a regenerative environment [15].
2. Proliferation Phase
Stem cells promote angiogenesis by secreting pro-angiogenic factors like VEGF (vascular endothelial growth factor) [14]. They also stimulate fibroblasts to produce extracellular matrix components, which are crucial for tissue regeneration [14].
Stem cells promote angiogenesis by secreting pro-angiogenic factors like VEGF (vascular endothelial growth factor) [14]. They also stimulate fibroblasts to produce extracellular matrix components, which are crucial for tissue regeneration [14].
3. Remodeling Phase
Stem cells enhance collagen synthesis and deposition, leading to better wound strength and reduced scarring16. Re-epithelialization also occurs, as stem cells aid in the migration and proliferation of keratinocytes, which are essential for the formation of new skin layers [16].
Stem cells enhance collagen synthesis and deposition, leading to better wound strength and reduced scarring16. Re-epithelialization also occurs, as stem cells aid in the migration and proliferation of keratinocytes, which are essential for the formation of new skin layers [16].
4. Cellular Dynamics
The plasticity of stem cells allows them to differentiate into various cell types needed for tissue repair, including keratinocytes, endothelial cells, and fibroblasts17. Stem cells migrate to the wound site in response to chemotactic signals, ensuring they reach the areas where they are most needed [17]. There, they can secrete growth factors like vascular endothelial growth factor (VEGF), fibroblast growth factor (FGF), and hepatocyte growth factor (HGF) that are crucial for angiogenesis and tissue repair13. Stem cell activity can be identified with surface markers such as CD73, CD90, and CD105 [18].
The plasticity of stem cells allows them to differentiate into various cell types needed for tissue repair, including keratinocytes, endothelial cells, and fibroblasts17. Stem cells migrate to the wound site in response to chemotactic signals, ensuring they reach the areas where they are most needed [17]. There, they can secrete growth factors like vascular endothelial growth factor (VEGF), fibroblast growth factor (FGF), and hepatocyte growth factor (HGF) that are crucial for angiogenesis and tissue repair13. Stem cell activity can be identified with surface markers such as CD73, CD90, and CD105 [18].
5. Treatments
Different types of treatments are used to ensure persistent efficacy of stem cell therapies, including:
Different types of treatments are used to ensure persistent efficacy of stem cell therapies, including:
Combination Therapies: Using MSCs with other treatments like plasma-rich proteins or scaffolds to enhance their efficacy [19].
Biophysical Modalities: Techniques like electrical stimulation and shock wave therapy to promote faster wound regeneration [12].
Engineered Skin Substitutes: Seeding MSCs on scaffolds or matrices to create skin substitutes that can replace damaged tissue [12].
6. Challenges and Considerations
A reliable and effective source of stem cells is required for these processes. Different sources like adipose tissue, bone marrow, and induced pluripotent stem cells (iPSCs) have varying efficacies and challenges [13]. One must also consider the most effective delivery method. These include topical application, scaffold incorporation, and combination with plasma-rich proteins [13]. These mechanisms collectively contribute to improved wound healing outcomes, making stem cell therapy a promising approach in regenerative medicine.
A reliable and effective source of stem cells is required for these processes. Different sources like adipose tissue, bone marrow, and induced pluripotent stem cells (iPSCs) have varying efficacies and challenges [13]. One must also consider the most effective delivery method. These include topical application, scaffold incorporation, and combination with plasma-rich proteins [13]. These mechanisms collectively contribute to improved wound healing outcomes, making stem cell therapy a promising approach in regenerative medicine.
The potential of using stem cell therapies in treating chronic wounds shows great promise across multiple studies and different cell types. Key findings from these studies include accelerated wound healing, reduced pain, and lower amputation rates. There are caveats though, the study using the CXCR4 antagonist demonstrated the complexity in stem cell mobilization. The overall trend is encouraging, but the small sample sizes, lack of blinding, and relatively short follow up periods in many studies underscore the need for larger, blinded, randomized controlled trials. Additionally, the variability in stem cell sources, preparation methods, and delivery techniques across the studies makes it challenging to draw definitive conclusions about what is the optimal approach. The body of research represents a significant step forward but also highlights the critical need for continued clinical trials in this promising area of medicine.
References
- Clayton, Z. E., Tan, R. P., Miravet, M. M., Lennartsson, K., Cooke, J. P., Bursill, C. A., Wise, S. G., & Patel, S. (2018). Induced pluripotent stem cell-derived endothelial cells promote angiogenesis and accelerate wound closure in a murine excisional wound healing model. Bioscience Reports. 38(4).
- Miao, Z., Jin, J., Chen, L., Zhu, J., Huang, W., Zhao, J., Qian, H., & Zhang, X. (2006). Isolation of mesenchymal stem cells from human placenta: Comparison with human bone marrow mesenchymal stem cells. Cell Biology International, 30(9): 681–687.
- Chen, L., Tredget, E. E., Wu, P. Y., & Wu, Y. (2008). Paracrine factors of mesenchymal stem cells recruit macrophages and endothelial lineage cells and enhance wound healing. PLoS ONE, 3(4).
- Kim, K. L., Song, S.-H., Choi, K.-S., & Suh, W. (2013). Cooperation of endothelial and smooth muscle cells derived from human induced pluripotent stem cells enhances neovascularization in dermal wounds. Tissue Engineering Part A, 19(21–22): 2478–2485.
- Loretelli, C., Ben Nasr, M., Giatsidis, G., Bassi, R., Lancerotto, L., D’Addio, F., Valderrama-Vasquez, A., Scherer, S. S., Salvatore, L., Madaghiele, M., Abdelsalam, A., Ippolito, E., Assi, E., Usuelli, V., El Essawy, B., Sannino, A., Pietramaggiori, G., Zuccotti, G. V., Orgill, D. P., & Fiorina, P. (2020). Embryonic stem cell extracts improve wound healing in diabetic mice. Acta Diabetologica, 57(7): 883–890.
- Hashemi, S., Mohammadi, A. A., Kabiri, H., Hashempoor, M. R., Mahmoodi, M., Amini, M., & Mehrabani, D. (2019). The healing effect of Wharton’s Jelly Stem Cells seeded on biological scaffold in chronic skin ulcers: A randomized clinical trial. Journal of Cosmetic Dermatology, 18(6): 1961–1967.
- Kirana, S., Stratmann, B., Prante, C., Prohaska, W., Koerperich, H., Lammers, D., Gastens, M. H., Quast, T., Negrean, M., Stirban, O. A., Nandrean, S. G., Götting, C., Minartz, P., Kleesiek, K., & Tschoepe, D. (2012). Autologous stem cell therapy in the treatment of limb ischaemia induced chronic tissue ulcers of diabetic foot patients. International Journal of Clinical Practice, 66(4): 384–393.
- Lu, D., Jiang, Y., Deng, W., Zhang, Y., Liang, Z., Wu, Q., Jiang, X., Zhang, L., Gao, F., Cao, Y., Chen, B., & Xue, Y. (2019). Long-term outcomes of BMMSC compared with BMMNC for treatment of critical limb ischemia and foot ulcer in patients with diabetes. Cell Transplantation, 28(5) : 645–652.
- Xu, S.-M., & Liang, T. (2015). Clinical observation of the application of autologous peripheral blood stem cell transplantation for the treatment of diabetic foot gangrene. Experimental and Therapeutic Medicine, 11(1): 283–288.
- Bonora, B. M., Cappellari, R., Mazzucato, M., Rigato, M., Grasso, M., Menegolo, M., Bruttocao, A., Avogaro, A., & Fadini, G. P. (2020). Stem cell mobilization with plerixafor and healing of diabetic ischemic wounds: A phase IIA, randomized, double-blind, placebo-controlled trial. Stem Cells Translational Medicine, 9(9): 965–973.
- Zollino, I., Campioni, D., Sibilla, M. G., Tessari, M., Malagoni, A. M., & Zamboni, P. (2019a). A phase II randomized clinical trial for the treatment of recalcitrant chronic leg ulcers using centrifuged adipose tissue containing progenitor cells. Cytotherapy, 21(2): 200–211.
- Zhao, Y., Wang, M., Liang, F. et al. (2021). Recent strategies for enhancing the therapeutic efficacy of stem cells in wound healing. Stem Cell Res Ther 12: 588.
- Nourian Dehkordi, A., Mirahmadi Babaheydari, F., Chehelgerdi, M. et al. (2019). Skin tissue engineering: wound healing based on stem-cell-based therapeutic strategies. Stem Cell Res Ther 10: 111.
- Farabi, B., Roster, K., Hirani, R., et al. (2024). The Efficacy of Stem Cells in Wound Healing: A Systematic Review. International Journal of Molecular Sciences. 25(5): 3006.
- El-Sayed, M.E., Atwa, A., Sofy, A.R. et al. (2024). Mesenchymal stem cell transplantation in burn wound healing: uncovering the mechanisms of local regeneration and tissue repair. Histochem Cell Biol 161: 165–181.
- Dekoninck, S., Blanpain, C. (2019). Stem cell dynamics, migration and plasticity during wound healing. Nat Cell Biol 21: 18–24.
- Margiana, R., Markov, A., Zekiy, A.O. et al. (2022). Clinical application of mesenchymal stem cell in regenerative medicine: a narrative review. Stem Cell Res Ther 13: 366.
- Grada, A., Falanga, V. (2018). Stem Cell Therapies for Wound Healing. In: Shiffman, M., Low, M. (eds) Chronic Wounds, Wound Dressings and Wound Healing. Recent Clinical Techniques, Results, and Research in Wounds, vol 6. Springer, Cham.
- Telci, D., Ilter, A.Z., Erdem, M. (2013). Stem Cells in Wound Healing. In: Turksen, K. (eds) Stem Cells: Current Challenges and New Directions. Stem Cell Biology and Regenerative Medicine. Humana Press, New York, NY.
Citation: Timothy Allen, Nepton Sheikhkoni, Andres Felipe Gonzalez, Jonathan Arnon, Ariella Allen, William Moradi and Lourdes Osado. (2024). “Stem Cell Therapies for Chronic Wound Management: A Comprehensive Review”. Journal of Medicine and Surgical Sciences 6.1.
Copyright: © 2024 Timothy Allen. This is an open-access article distributed under the terms of the Creative Commons Attribution License, which permits unrestricted use, distribution, and reproduction in any medium, provided the original author and source are credited.